Little is known about the important transitional period from about 2.0 billion years ago until about 0.7 billion years ago.
-- Robert Garrels
If you are curious about the Earth and wonder about the history of rocks, there are few better places to be born than England or Wales. My small island, which lies within both of these countries, has as many geological periods as a continent. Along its lengthy shoreline the waves have cut cliffs and these walls of rock display their dissected strata as in a museum diorama. I used to spend childhood holidays at a place called Chapman's Pool on the coast of the county of Dorset; here the somber black cliffs of Kimmeridge shale were speckled with snow-white ammonites and other fossils.
As you move westward across England the rocks go back in history, and by the time you come to Wales their age approaches 570 million years. These old rocks are called Cambrian, after the Roman name for Wales. They are the oldest to bear fossils visible to the unaided eye. There are, of course, older rocks containing microfossils of bacteria, such as those that Barghoorn and Tyler found; but before modern methods of dating there was no sure way of knowing their age. The period with rocks older than those bearing the larger fossils was called Precambrian, because it was further distant in time than the rocks of Cambria. We now know that the Precambrian has parts that are very old indeed. This new knowledge comes from the distribution in these old rocks of the radioactive elements uranium and potassium, and their products, lead and argon. Radioactive decay is an accurate clock; by measuring the proportion of uranium to lead or of potassium to argon in a piece of rock its age can be calculated. Other evidence about ancient rocks comes from the distribution of the isotopes of that stable element, carbon. This, and the discovery of Archean bacterial microfossils, tell us that life was present at least 3.6 eons ago. The Precambrian is now mapped and divided into the Proterozoic period, 0.57 to 2.5 eons before now, and the Archean, 2.5 to about 4.5 eons. Some geologists call the first period, 4.5 to 3.8 eons, the Hadean.
Like the Archean, the Proterozoic was a time when the ecosystems of the Earth were populated by bacteria (the prokaryotes). In the anoxic regions of the sediments the Archean bacteria would have lived on; but in the now mildly oxidizing ocean and surface environments there eventually developed more complicated living cells, the eukaryotes. These are the ancestors of large communities of nucleated cells, like the trees and ourselves.
The Proterozoic is still an enigmatic period of the Earth's history. I feel free, therefore, to use it as a background on which to develop geophysiological models of what it might have been. I shall write this chapter not as history but as an account of the physiology of an unknown animal who lived thousands of years ago, drawn from no more evidence than a few scraps of bone accurately dated from their content of carbon isotopes. My main interest is in the long-term geophysiological processes that kept the Earth constant and fit for life, and in how they worked. With the bones of the Earth in mind, I shall keep returning in thought to that important element, calcium, and its crucial role in all living things from ourselves to Gaia. I shall continue to be concerned with those other important elements, oxygen, carbon, and hydrogen, and with their regulation and with the climate. This chapter will also be about the geophysiology of the oceans; in particular the difficult problem of whether the total salinity is determined by physical and chemical forces alone, or whether there is "machination" on the part of Gaia. Although the setting of the chapter is the Proterozoic, the middle ages of the Earth, most of the topics discussed are not unique to that period; they acted also in the Archean, and continue to act in the present period.
If we aim to start at the boundary between the Archean and the Proterozoic, we shall find that this boundary is still under negotiation. There is no clear-cut frontier, just a no man's land where field geologists set their posts according to their fancy. The Archean geologist Euan Nisbet tells me that there is an informal acceptance of the date 2.5 eons before the present time, although some would prefer to set the fence at the date marked by the appearance of a special suite of rock in Zimbabwe. Just as political frontiers often fail to circumscribe ethnic regions accurately, so boundaries based on geological considerations alone do not always suit the interests of geophysiology. As a geophysiologist, I prefer to set my markers at the time when the environment became predominantly oxidizing -- or to put it more professionally, at the transition from an environment dominated by electron donor molecules, like methane, to one dominated by electron acceptors, like oxygen. As it happens, the uncertainties about the events 2.5 eons ago are large, and for the time being we can take the geological and the geophysical markers to define the same zone of the Earth's history.
For geophysiology, the important thing about the transition from the Archean to the Proterozoic is not the exact date of the event, but that it happened. It is rather like puberty; a profound physiological change but one spread over a finite period of time. In puberty markers of the change -- the appearance of the beard and the deepening of the voice, or the expansion of the breasts -- are secondary to the main event. The switching on of these secondary sex characteristics is the response to an increasing flux of pituitary hormone. This primary event may be sharply defined but the secondary characteristics are somewhat arbitrarily scattered in time. Between the Archean and Proterozoic the appearance of oxygen as a dominant atmospheric gas was the primary event and marked a profound change in the Earth's geophysiological state. The outward and secondary manifestations of this change -- the emergence of a new surface and atmospheric chemistry and of ecosystems as oxygen began to dominate the atmosphere -- is likely to have spanned a significant interval of time, and happened at different times in different places.
From early in the Proterozoic to the present day there has been an excess of free oxygen gas in the air. By an excess, I mean that the atmosphere has carried more oxygen than is needed to oxidize completely the short-lived reducing gases: methane, hydrogen, and ammonia. When the Proterozoic began, geophysiologically speaking, the division of the two great planetary ecosystems, the oxic surface regions and the anoxic sediments, was complete. The Archean, when the environment was full of molecules that donated electrons (that is, reducing agents), did not so much end as become encapsulated as a separate region that exists wherever oxygen is absent. The submission of the anoxic ecosystems to domination by the oxic was somewhat like the Norman conquest with the Archean Saxons driven to a subservient underground position -- the lower classes -- from which, it is often said, they have never escaped.
The change from anoxic to oxic was a crucial step in the Earth's history. In the model of the Archean in the previous chapter (figure 4.2), the end of the period was pictured as very sudden, with oxygen rising from very low to between 0.1 and 1 percent atmospheric abundance in not more than one million years. This is, of course, no more than the prediction of a geophysiological model; one that sees the change from one regime to another as an event driven by powerful positive feedback from the biota and the environment.
The conspicuous difference between the Archean and the Proterozoic is, I think, in the composition of the atmosphere and the oceans; and possibly also in the climate. The simple model in figure 4.2 supposed that the carbon cycle was preserved by the methanogens which returned a massive flux of methane and carbon dioxide to the air from the sediments, and that this state persisted until, quite suddenly, free oxygen appeared. More probably there was some oxygen present even early in the Archean, just as there is methane in our present atmosphere. The difference between the Archean air and the Proterozoic air was not a simple matter of the presence or absence of oxygen, it was in the net tendency. In the Proterozoic, a discarded bicycle left in shallow water would have rusted away to form insoluble ferric oxide which settled on the sea floor; in the Archean, it would have slowly dissolved as water-soluble ferrous iron, and left no trace. During the time that the flux of methane exceeded the flux of oxygen, the lower atmosphere could carry only trace quantities of oxygen. The oceans and surface rocks, rich in the oxygen-scavenging ferrous iron and sulfides, would have absorbed so large a proportion of the oxygen output of the cyanobacteria that the air would have remained in a net anoxic state for most of the Archean period.
We do not know that the oxygen of the air rose suddenly; it may have risen slowly or in a series of steps. It is important also to distinguish between the presence, and the dominance, of oxygen; dominance, in a chemical sense, requires that the ratio of oxygen to methane is greater than two to one. The reason for thinking that the change of regimes, the boundary of the Archean and Proterozoic periods, was abrupt is the evidence of a major glaciation at about 2.3 eons ago. This might have come as a result of a sudden fall in atmospheric methane. Such an event would be accompanied by cooling, since methane and its decomposition products are greenhouse gases. There are also geophysiological arguments that favor a sharply defined transition to an oxidizing state. Once the photosynthetic oxygen became dominant in the atmosphere and oceans, the action of sunlight on oxygen would produce hydroxyl radicals that oxidize the methane in the air. Also, there would be consumers feeding on the organic matter before it could reach the anoxic sediments; this would deny to the methanogens the material for the production of their gaseous excretion. This is the recipe for a powerful positive feedback against methane and in favor of oxygen. These events are likely to have been sudden rather than gradual. Finally, there is the ecology to take into account. There would be ecosystems existing in the Archean that found their world comfortable. As they evolved with their environment, they would resist change, but their resistance would be like that of a locked fault in an earthquake zone. They would tend to resist change, trying to keep the status quo, but when the break came it would be all the more sudden and devastating.
The transition to oxygen dominance may have left its mark in the record of the rocks in the form of the Gowganda glaciation, but the long subsequent period is one of the more obscure periods of the Earth's history. Gaia theory requires that the tightly coupled evolution of living organisms and their material environment will have determined the state of the Earth in this as in the other periods. Can we envisage from this theory a living planet existing in the Proterozoic, and the regulatory systems that may have operated then?
When Earth scientists use the word regulation, they usually have in mind a passive process where the input and output of some component or property are in balance. In geophysiology, by contrast, regulation implies the active process of homeostasis; the preservation of a comfortable Earth by the interaction of life and its environment. The speculations that follow about the regulation of climate, oxygen, salinity and other properties of the environment are in this geophysiological context, in other words, as if they were speculations about the state of a living organism. In no sense is this intended as a teleology, or meant to imply that the biota use foresight or planning in the regulation of the Earth. What we need to think about is how a global regulatory system can develop from the local activity of organisms. It is by no means far-fetched to imagine a single new bacterium evolving with its environment to form a system that can change the Earth. Indeed the first cyanobacterium, progenitor of the ecosystem that used light energy to make organic matter and oxygen, did just this.
If the element oxygen was crucial in the geophysiological evolution of the atmosphere, then calcium must surely be the determining element in the geophysiology of the oceans and the crust. Calcium is one of the alkaline-earth elements occupying the second column of Mendeleev's famous periodic table of the elements. It comes after magnesium and before strontium. It is the third most abundant positive ion of sea water, after sodium and magnesium. We tend to think of calcium as a benign and nutritious element because it is an essential structural component of our bones and teeth. It is also crucial in numerous internal physiological processes from blood clotting to the division of cells. It is essential for life but, paradoxically, it is very toxic in the free ionic state. Within our cells a concentration of calcium ions exceeding a few parts per million is lethal, comparable in toxicity to cyanide, yet calcium ions are free in the oceans at a level ten thousand times greater.
In chapters 2 and 3, I explained the operation of the Daisyworld model in terms of the competitive growth of organisms when one environmental property is sharply circumscribed. Too much and too little are both uncomfortable; there is a preferred state between torrid heat and freezing cold, between excess and starvation. This is particularly true of calcium. Imagine some bacterium in the early oceans able to convert the abundant water-soluble calcium ions of its internal environment into insoluble calcium carbonate. This simple reaction would have effectively reduced the concentration of the potentially toxic calcium ions within the cell, by locking up calcium in a safe insoluble form. Such an action, if calcium were in excess as it usually is in the oceans, would have increased the organism's chances of survival and those of its progeny. These organisms would be at an advantage compared with organisms that merely adapted to the presence of excess calcium. In the sunlit zone of the open ocean, the growth of these organisms would lead to vast masses of calcium carbonate being deposited on the ocean floor. The rain of microscopic "sea shells," called tests by marine biologists, from the sunlit surface to the depths acts like a conveyor belt. Food is brought for consumers lower down, the ocean is swept clear and made transparent, and potentially toxic elements, like cadmium, are taken from the surface regions. Carbon dioxide and calcium are transported, and assembled together by bacterial communities to form the flat or mushroom-shaped rock cities called stromatolites. The concentration of calcium ions in the oceans would have been reduced, and all life would have flourished as a consequence. The ubiquity of limestone deposits of oceanic origin implies the success and continuation of this activity. In contradiction to this view, some geologists believe that early limestone deposition was an inorganic process. I do not see how we can distinguish between the spontaneous crystallization of super-saturated calcium carbonate in the Archean and nucleation induced by organisms. I do think that the nucleation of supersaturated and other metastable states in Nature is a key geophysiological process, and that it originated in the Archean.
I know as an inventor that really good inventions tend to grow and evolve. Only weak inventions take a single step and no more. Consider how the simple semiconducting crystal of those first radio receivers in the 1920s has evolved to become, in a grand eutrophication, the ubiquitous silicon devices of today. The calcium carbonate precipitation step was an even greater invention; it led not merely to the regulation of calcium, carbon dioxide, and climate but also to the vast engineering of the calcium carbonate structures (the stromatolites). Later, these same processes evolved so that our own cells possess intricate mechanisms by which calcium is deposited as bones and teeth.
Most remarkable of all, biological calcium carbonate deposition may have made possible the efficient operation of the endogenic cycle -- the slow movement of the elements from the surface and the ocean to the crustal rocks and back again. The geologist Don Anderson has speculated that the deposition of limestone on the ocean floor is a key factor in the motion of the Earth's crust. He proposed that sometime far back in the Earth's history, sufficient limestone was deposited to alter the chemical composition of the crustal rocks of the ocean floor near the continental margins. As a result an event, called the basalt-eclogite phase transition by geologists, took place. This transition so altered the physical properties of the crustal rocks that it became possible for the great machinery of plate movement to begin turning. Don Anderson commented in an article in Science in 1984:
The Earth is apparently also exceptional in having active plate tectonics. If the carbon dioxide in the atmosphere of Venus could turn into limestone, the surface temperature and those of the upper mantle would drop. The basalt-eclogite phase change would migrate to shallow depths, causing the lower part of the crust to become unstable. Thus there is the interesting possibility that plate tectonics may exist on the Earth because limestone-generating life evolved here.
To me this is an exciting idea, but I admit that most geologists find it extremely improbable. The event may have begun with the activity of a few organisms able to split a dilute solution of calcium bicarbonate into chalk and carbon dioxide, and so avoid calcium poisoning. We do not know when plate tectonics started. If it is connected with life, that connection may not have existed before the development of the intracellular precipitation of calcium carbonate in eukaryotes, late in the Proterozoic.
The study of the intricate biological processes for segregating and concentrating the elements of the crust and ocean in the form of minerals has become a separate topic of the Earth sciences called biomineralization.
Salt regulation is one of the most interesting and tantalizing Gaian systems. There are few organisms able to tolerate salt at concentrations above about 6 percent by weight. Have the oceans always kept below this critical limit of salinity by chance? Or has the tightly coupled evolution of life and the environment led to the automatic regulation of ocean salinity? It is often stated that the preferred internal saline medium of living things, one that is astonishingly similar over a very wide range of organisms, reflects the composition of the oceans when life started. It is true that the salinity of the blood of whales, humans, mice, and of most fish, whether dwelling in the ocean or in fresh water, is the same. Even the circulating fluid of Artemia, the brine shrimp that lives in saturated salt solutions, shares with us the same internal salinity. But to my mind this is no more evidence of the salinity of the Archean ocean than are the oxygen levels now breathed by these organisms evidence of the oxygen abundance at the start of life.
Most cells survive and do best in a medium whose salinity is 0.16 molar (about one percent by weight in water, or normal saline). Many kinds of cells survive the salinity of sea water, 0.6 molar, but above 0.8 molar the membranes that hold the precious interior contents of cells become permeable or disintegrate completely. The reason for the destructive action of salt solutions is simple. Cell membranes are held together by the same kind of forces that hold together a soap bubble. Quite often these forces are very sensitive to the salinity of the medium, usually being weakened when the salinity is high. You can see this for yourself by making bubbles with increasingly strong salt solution as the solvent for the soap. Above about 10 percent salt, bubbles cannot be made. This is because soap molecules are made up of a long chain of carbon atoms tightly linked together and surrounded by hydrogen atoms equally firmly held. These chains are terminated at one end only by a carbon atom with two oxygen atoms attached. When this end of the soap molecule is dissolved in water that is slightly alkaline, as all soap solutions are, it carries a negative charge. The negative electrical charge makes the end group attract water molecules and drags the insoluble oily hydrocarbon chain into solution. Salt is made up from negative chlorine ions and positive sodium ions. When there are many of these in solution they begin to compete with the soap's negative charge for the water molecules. When enough salt is present, the soap separates from the water as curd.
The molecules of a cell membrane are more complex than soap: they include such substances as sterols (for example, cholesterol), hydrocarbons, proteins, and phosphatides. The molecules of phosphatides correspond to those of soap, and are the part of the membrane most affected by an increase in salt concentration. High salt concentrations disrupt cell membranes by disturbing the electrical forces holding the membrane in its correct and complex state. The membrane of the human red blood cell, for example, is made up of more or less equal parts of cholesterol and lecithin (a phosphatide) and a mixture of protein and other fatty substances. The red blood cell will survive exposure to salt solutions up to 0.8 molar (4.7 percent by weight in water). Above this strength the membrane is damaged, and at concentrations above 2.0 molar the cell may be destroyed in seconds. In the mid-1950s I was able to show by direct experiments that the damage to red blood cells commenced when lecithin dissolved away from the membrane into the strong salt solution. This kind of salt damage seems general among living cells and is seen with the cells of all five kingdoms.
The salt concentration of today's sea is always uncomfortably high for living organisms. The larger ones, such as fish, swimming mammals, and some crustacea, have physiological mechanisms to regulate the internal salt at a level close to that of their own bodies (0.16 molar). To prevent the loss of water from their internal medium (osmosis), these animals have to work to stop themselves being squeezed dry by the osmotic pressure. They have to use energy to pump water in against the osmotic pressure difference between their interior and that of the sea. The pressure is close to that needed to pump water 450 feet vertically against gravity, over a thousand times the blood pressure of humans. The advantages of a low-salt interior must be considerable to require such an effort to sustain it. For the small cell of a bacterium, individual regulation is a luxury far beyond its means. This does not just apply to salinity. Take temperature for example: for a microorganism to sustain even a 1°C difference from that of its environment would require the consumption of far more food and oxygen than could cross its surface. Not only this, but a 1° temperature gradient across the cell membrane would generate a thermal osmotic pressure of 56 atmospheres, or 840 pounds per square inch; far beyond the strength of a cell membrane to resist.
The most frequent experience of salt stress is in drying or freezing. When cells freeze, water is removed as pure ice and the salt solution in which they exist becomes concentrated. Freezing and drying must have been common hazards from the beginning of life, and indeed no direct answer to this problem of salt damage to membranes has evolved in all the time since then. There are salt-tolerant bacteria, the halophiles, that live precariously in the saline regions of the Earth. These bacteria have solved the problem directly by evolving a special membrane structure that is not disrupted by salt. It works, but at a price; for these organisms cannot compete with mainstream bacteria when the salinity is normal. They are limited to their remote and rare niche, and depend upon the rest of life to keep the Earth comfortable for them. They are like those eccentrics of our own society whose survival depends upon the sustenance that we can spare but who could barely survive alone.
Mainstream life, therefore, is limited to a maximum salt concentration of about 0.8 molar. This is not much greater than the saltier parts of the ocean, where the salinity reaches 0.68 molar. It is frequently exceeded whenever the tide recedes, leaving organisms soaked in sea water to dry out on the shore. The problem of salinity must have arisen for the Archean bacteria. Their answer to the problem was to synthesize soluble compounds called the sulfur and nitrogen betaines. They make these neutral solutes, which substitute for the salt and are not toxic to the cell. When these are present in the cells and their medium, freezing or drying no longer concentrate the salt to destructive levels. Even so, there is a price to pay for the synthesis of these anti-salt betaines. As much as 15 percent of the dry weight of shoreline algae is betaine; a considerable diversion of the energy that would otherwise be available to the organisms. Clearly, it would be to the advantage of the biota to keep the oceans as dilute as possible -- certainly to keep them from approaching the critical 0.8 molar concentration.
Problems of salt regulation exceed in difficulty anything humankind has so far done in the way of planetary engineering. The Archean bacteria could relatively easily modify that small compartment, the atmosphere, to suit their needs; but the vast mass of the oceans, some ten thousand times larger, was very much more difficult to manipulate. The only way to remove the huge masses of salt in the oceans would be to segregate ocean water in lagoons and allow the sun's heat to evaporate the water away. It would have required the building of vast limestone reefs to trap salt in evaporite lagoons. The sheer magnitude of these reefs would dwarf any conceivable human construction. It may even be possible that the process of lagoon formation was assisted by the folding of rocks at the continental edge as a consequence of the movement of the plates.
Table 5.1 SALTS OF THE OCEANS
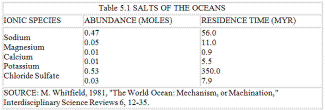
Table 5.1 lists the salts of the oceans. Salt in solution, weathered from the rocks, is entering continuously from the rivers and also from the interior of the Earth at the sea-floor spreading zones that lie at the bottom of all the major oceans. In the ocean, salt is not a single substance, sodium chloride. Rather, it exists as positive sodium and negative chlorine ions, and these behave as two quite independent and separate entities. The sodium ion and the other positive ions of potassium, magnesium, and calcium all have relatively short residence times in the ocean. They are removed by biochemical and chemical processes, and also by hydrothermal chemical reactions within the sea-floor spreading zones, and deposited as sediments, clays, limestones, and dolomite. The salt problem for the biota is actually a problem of getting rid of the negative chloride ions.
Chemically, the chloride ion is rather like an atom of the wholly unreactive gas argon. It is a smooth, slippery, spherical molecule with little or no tendency to attach to anything. There is no significant biochemical trade in chlorine. A few strange systems do make methyl chloride from salt, but the turnover of this compound is too small to affect the salinity of the ocean. Also, the chlorine in methyl chloride soon becomes chloride ions again, and is washed out by the rain and returns to the sea. Chloride ions are removed from the oceans physically by the transfer of sea water to the evaporite lagoons. The water trapped in these lagoons warms in the sun and evaporates; the water vapor moves through the atmosphere and eventually condenses elsewhere as rain -- pure water -- that flows into and dilutes the ocean. The salt, represented by the dominant chloride ion and the positive ions that must go with it to keep the total ionic charge zero, is left behind as crystalline layers. These evaporite lagoons are found in many places on the continental margins. Fossil lagoons exist in many places beneath the Earth's surface, sometimes even beneath the ocean itself.
Before these lagoons can form, barriers are needed at their seaward boundary. Could this activity be part of the tightly coupled evolution of life and the rocks, or is it just the result of chance? The key process in the formation of these barriers is the deposition of calcium carbonate. The carbon dioxide in the air reacts continuously with alkaline rocks on the land surfaces to form bicarbonates. An important reaction of this type is the one between calcium silicate rock and carbon dioxide dissolved in surface water. The product is a solution of silicic acid and calcium bicarbonate, which flows down the rivers to the ocean. In the absence of life, the calcium and bicarbonate ions can coexist in a mildly acid ocean, and the continuous supply of these would eventually lead to the spontaneous crystalization of calcium carbonate. But it would be more or less randomly deposited over the ocean floor. Limestone deposits in the real world are mostly from the action of living organisms. Limestone is not deposited either randomly or according to the expectations of physics and chemistry. The precipitation of calcium carbonate by colonies of microorganisms occurs most extensively in the shallow waters around the continental margins where the abundance of both nutrients and calcium bicarbonate are highest. Without any planning or foresight, the components of those living structures, the limestone stromatolites, would have assembled offshore and eventually sealed off lagoons from which sea water would be progressively evaporated and salt deposited. At first the reef building would have only a local effect, but over time the sheer mass of the limestone would begin to affect the plastic crust of the Earth's surface, depressing it and so extending the size of the lagoon. New rock formers would always be colonizing the surface of a reef as it descended, so tending to keep the lagoon intact. If, as Don Anderson has suggested, the motion of the Earth's crust depends on the continued deposition of calcium carbonate in the sea, the limestone reefs could have led to the complex events of mountain building and the folding of rocks at the continental margins. This, in turn, would extend the range of shorelines where evaporite lagoons could form.
During the course of time salt is being added to the oceans from the lithosphere and removed again. Some of this salt is deposited in evaporite beds and buried beneath sediments. These deposits may be a temporary store which Earth movements and weathering continuously expose and release the contents of back to the sea; but new evaporite lagoons are always forming. The balance of erosion and formation seems always to have kept enough salt sequestered in evaporite beds to keep the oceans fresh and fit for life. The evidence that lagoon formation and maintenance depends on the specific behavior of marine micro' organisms is strong.
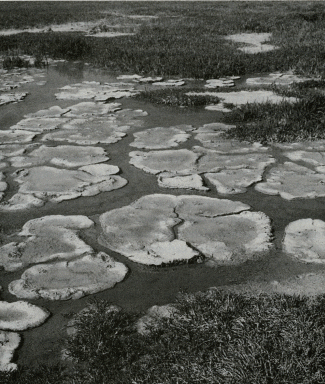
5.1 The evaporite lagoons at Laguna Figueroa, Baja California, Mexico. Sand dunes form a barrier through which sea water percolates. The water then evaporates in the lagoons, and salt crystallizes out to form an evaporite deposit. The surface of the lagoons are often covered by microbial cell communities called mats.
I once had the pleasure of joining an expedition, led by Lynn Margulis, to the microbial mat communities that form in the evaporite lagoons of Baja California in Mexico (as shown in figure 5.1). They are on the western edge of that long, narrow tongue of land that hangs down below San Diego and separates the Gulf of California from the Pacific Ocean. Here I was able to see at first hand the subtle economy of the bacterial mats that covered the lagoon. The red and green communities of microbes at the surface acted as a raincoat, preventing the salt from being dissolved in the rain and washing back into the ocean. Indeed, on one occasion, the whole lagoon was flooded by feet of fresh water. Within two years the flood was evaporated and dispersed without destroying either the microbial communities or the evaporite bed beneath. In normal times, the movement of rain water downward through the mat lowers its salinity and assists the growth of photosynthesizers at the surface, the primary providers of food and energy for the communities beneath. Salt crystals at, or their, the surface are also coated with their own specific varnish and protected against easy solution in rain water.
Is all this a grand, unplanned civil engineering enterprise by Gaia? The steps, from the individual lowering of calcium ions within the cells of a living organism to the movement of the plates, are all those that tend to improve the environment for the organisms responsible. But the links between biomineralization, salt stress, and plate tectonics are so tenuous that most scientists would think them to be connected by chance rather than by geophysiology. I shall continue to wonder about the limits of Gaian manipulation and always seek guidance by asking the simple question: What would the Earth have been like without life? Would limestones have precipitated at the continental margins so as to form evaporite lagoons? Would the salt have deposited in them, or would it have been washed back into the ocean by rain in the absence of a living raincoat of microbial mat? Would limestone have deposited at the sites and densities needed to start the plates moving? Unlikely perhaps, but remember there have been billions of years for geophysiological invention and its trial by natural selection. We should consider the possibility that this long period was sufficient to fine tune the rough geology into a smoothly regulated geophysiology.
So far we have been considering mainly the large-scale engineering works. What about the workers? During the Proterozoic, a new type of cell evolved, those with nuclei, called the eukaryotes. These are cells that contain structures within them, and other organelles (such as chloroplasts, the green-pigmented bodies that do the work of photosynthesis). Lynn Margulis has taught that these more complex cells are really communities of bacteria that once lived free but now are contained within the outer membrane of one of them. In her book Early Life she tells how the presence of oxygen in the Proterozoic set the scene for the appearance of these new and more powerful cells. It was an evolutional step like that which occurred in the Archean, when the ecosystem of photosynthesizers using carbon dioxide came to equilibrium with the methanogens that returned carbon to the atmosphere as methane and carbon dioxide.
Oxygen opened a giant new niche for organisms that could survive in it and use it. At first these new organisms, who gained energy by combining the organic matter with oxygen, may have existed peacefully with the photosynthesizers, merely eating their debris and dead bodies. But before long there would be consumers, organisms that had learnt to eat fresh food and that grazed the photosynthesizers as they grew. Cells do not have mouths, but they can ingest other cells by enclosing them within a pocket in their membrane, a process called phagocytosis. The pocket becomes part of the cell's interior and dissolves away, leaving the captive entrapped within. Digestion would be the normal fate, but sometimes the roles would be reversed and the ingested organism could be the aggressor. Tubercle and leprosy bacteria do this trick even today, attacking the phagocytes that ingest them instead of succumbing as victims to the phagocytes' powerful digestive system. The results of warfare, however, are rarely genocide; instead war can lead to a peaceful coexistence mutually beneficial to the victim and the aggressor. In this way, the chloroplasts have as ancestors the cyanobacteria of the Archean, and today they power the cell communities of cabbages and redwood trees. Although briefly discussed by nineteenth-century biologists, this powerful association of organelles working within cells in a process of symbiosis, called endosymbiosis, is recognized due to Lynn Margulis more than to anyone else. Endosymbionts enlarged and expanded the possibilities of planetary manipulation by the biota and were a main feature of the history of the Earth during the Proterozoic.
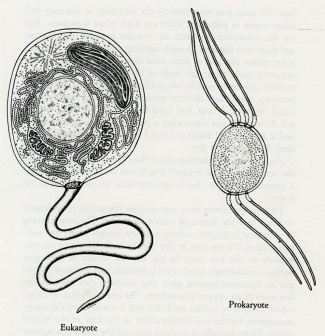
Eukaryote. Prokaryote.
5.2 Eukaryotic and prokaryotic cell structures compared. The eukaryotes differ in having membrane-bound organelles which include the nucleus, mitochondria, and chloroplasts. (Drawing by Christie Lyon.)
The formation of collectives gives power to the assembly greater than that possessed by its individual components; but this is never without a price. For the early bacteria (the prokaryotes), aging was not a problem. They had neither nucleus nor organelles, and they carried their genetic information on a few strands of DNA within the cell membrane (see figure 5.2). Genetic information lost by an individual bacterium during its brief life span was recovered by the exchange of plasmids and other pieces of polymeric software with other organisms. But for the eukaryotic cell with its complex internal organization and organelles (figure 5.2), each carrying a different set of genetic instructions, the loss of some vital item of information by one organelle could mean the death of the cell. The invention of a method and mechanism for the deliberate transfer of information between cells before division greatly reduced the chances of a lethal decision. It was this need that led to the invention of sex. It is much too interesting a story to try to abstract here, and is given in full detail in Lynn Margulis and Dorion Sagan's book The Origins of Sex.
An unanswered question about the Proterozoic is, What was the concentration of oxygen? Did it just stay at around 0.1 to 1 percent, or did it rise in concentration to present levels or higher?
Free oxygen comes from two sources: the escape of hydrogen to space and the burial of carbon or sulfur. The sequestering of elemental hydrogen, carbon, or sulfur always leaves free oxygen behind. As we saw in the previous chapter, once oxygen appears in the free state in the air, the escape of hydrogen becomes vanishingly small. This is because only traces of hydrogen or hydrogen-bearing gases like methane can exist in the free state in an oxygen atmosphere. The one exception is water, which cannot be further oxidized, and is confined to the lower atmosphere by the low temperatures at the base of the stratosphere. Quite literally, it is frozen out; and the upper atmosphere contains only a few parts per million of water vapor. The present rate of escape of hydrogen to space is limited by the dryness of the upper air and is only 300,000 tons a year. This is equivalent to just under 3 million tons of water, and would leave behind an excess of 2.5 million tons of oxygen. It sounds a lot, but a loss of water of that rate would have removed less than one percent of the oceans in the age of the Earth.
Once hydrogen loss was reduced to trivial significance, the only way to add more oxygen was to separate carbon and sulfur from combination with oxygen in carbon dioxide and sulfates. If the separated carbon and sulfur could be buried in the sediments before they had an opportunity to react again with oxygen, a net increment of this gas would be added to the air. This process of separation starts with photosynthesis, which splits carbon dioxide into oxygen, which then enters the air, and into the living and dead parts of the plants and bacteria. Most of this carbonaceous material is recombined with oxygen by consumers, but a little, about 0.1 percent, is buried more or less permanently. Some of the carbon in the sediments is used to reduce sulfate to sulfides. The burial of sulfides also leaves a net increment of oxygen in the air. The carbon and sulfides are buried in the sediments mixed with shales and limestones. The burial can take place in such a way as to form fossil fuels, coal and oil; but these represent only a small proportion of the total carbon and sulfur in the sediments. The burial of all the oxidizable material is like a loan drawn against the oxygen account. So long as it is buried or lost in the Earth's interior, the debt is not presented and free oxygen can remain in circulation in the air.
At present about 100 million tons of carbon are buried each year -- equivalent to the release of 266 million tons of free oxygen gas to the air. (This does not mean that oxygen of the atmosphere is increasing, for the increment is all used up by the oxidizable materials released by volcanoes, by weathering, and by processes at the sea floor.) The rate of carbon burial has been constant throughout the history of life on Earth; there is very little difference between the Archean and now. This is curious when you consider that the mass and the activity of the biota may have been less in the Archean. The puzzle can be solved if we remember that because there was only a trace of oxygen present, the proportion of oxic consumers to anaerobes would have been less than now. This means that the methanogens and other organisms of the anoxic sector were digesting nearly all the products of photosynthesis, but buried the same amount of carbon as now. The high rate of photosynthesis today must, in part, be due to the rapid recycling of carbon by the oxygen-breathing consumers. They metabolize 97.5 percent of the products from photosynthesis, leaving only 2.5 percent for the anaerobes. In the Proterozoic, there were consumers present feeding on organic matter and using oxygen to metabolize it. Their activity is likely to have been less than now but greater than in the Archean.
The key point is that oxygen production is determined by the amount of carbon buried, and this in turn depends upon the proportion of the products from photosynthesizers that reach the anoxic sector. Obviously, if the consumers eat all the organic matter there would be none left to be buried and, therefore, no source of oxygen. If we recall that the rate of carbon burial has been more or less constant, then it follows that the input of oxygen from this source has also been constant. In the Archean all this oxygen went to oxidize the reducing substances in, and being added to, the environment, but when free oxygen appeared an increasing proportion of it was used by consumers. The continued existence of the Archean anoxic ecosystems ensured the continuous burial of carbon and a continued input of oxygen to the air. These possibilities are summarized in table 6.1. What, then, determined the oxygen level of the air? Arguing from geophysiology, we can suppose that the inherent toxicity of oxygen is not entirely overcome by the antioxidant systems and by the enzymes of the organisms of the oxic sector; in these circumstances oxygen may set its own limit. Like temperature, it would be an environmental property with a lower and an upper limit for mainstream life. Such properties can be geophysiologically regulated.
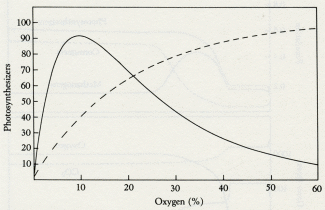
Oxygen (%)
5.3 The effect of oxygen on the growth of organisms (solid line) and the effect of the presence of organisms on the abundance of oxygen (dashed line). Where the two curves intersect is the level of oxygen at which the system regulates.
Figures 5.3 and 5.4 illustrate how it might have been done in the Proterozoic. Figure 5.3 depicts the effects of oxygen on the growth of the oxic ecosystem and the effect of the size of the oxic ecosystem on oxygen. The solid line is the relationship between a steady level of oxygen and the population of oxygen-using consumers; at low oxygen levels they could not metabolize and at high levels they would be poisoned by oxygen. The dashed line indicates the relationship between the population of the oxic ecosystem and the steady-state level of oxygen; the more photosynthesizers, the more oxygen. The two curves intersect at an oxygen level that would be kept in homeostasis by the system.
Figure 5.4 illustrates the calculations of a computer model where photosynthesizers, consumers, and anaerobes coexist on a planet before, during, and after the appearance of oxygen. It is assumed that, as on the Earth, the constant burial of carbon and a declining turnover of reducing rocks and gases oxidizes the planet until free oxygen becomes a dominant gas. Thereafter oxygen rises until the geophysiological properties of the system establish a new steady level where the abundance of oxygen is kept constant by a balance between the quantity of carbon buried and the quantity of reducing material exposed. The lower panel illustrates the planetary temperature variations, in comparison with those of a lifeless planet of the same composition. The middle panel shows the variation of the oxygen, carbon dioxide, and methane gases. The upper panel shows the population levels of the different life forms. This model is a linear descendant of the climate models in chapters 2 and 3, where the competitive growth of differently colored daisies is shown to be capable of regulating the temperature of a model planet. It accepts that, in the long term, a constant amount of the carbon produced by photosynthesis is buried and that this source of oxygen is constant. The sink for oxygen would be declining. At the end of the Archean, oxygen rose in abundance. The presence of excess oxygen would have increased the rate of weathering and so increased the supply of nutrients, which in turn would have favored a larger ecosystem. More carbon would have been buried, and the rise in oxygen would have accelerated until toxicity began to set a limit. By this time, the anaerobic sector from which carbon burial takes place would have shrunk to the same size as in the Archean, and oxygen production would again equal oxygen loss by the exposure of oxidizable substances during weathering.
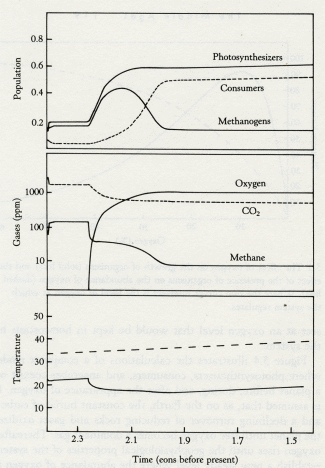
Time (eons before present)
5.4 Model of the transition from the Archean to the Proterozoic. The lower panel shows climate with a lifeless world (dashed line) compared with a live world (solid line). Note the sudden fall of temperature when oxygen appears. The middle panel shows the abundance of atmospheric gases (carbon dioxide, dashed line; oxygen and methane, solid lines). The upper panel illustrates the changes in population of the ecosystems as the transition is entered and passed. Note how both photosynthesizers and methanogens increase when oxygen first appears and how methanogens fall back to a steady level when the oxygen-breathing consumers (dashed line) become established.
In one sense the oxic ecosystems existed right from the beginning of Gaia; from the moment when the first cyanobacteria converted sunlight into high potential chemical energy and were able to make organic compounds and oxygen from water and carbon dioxide. As the cyanobacteria spread, they would always have occupied a surface position to enjoy and feed on the sunlight. The anoxic systems, whose food was the dead bodies and products of the cyanobacteria, would naturally have existed below the photosynthesizers to take advantage of the conveyance by fallout of the food from above. From the start, there would have been a segregation of these two ecosystems, and a gradient of oxygen concentration declining away from the region of its production.
In the real world, the oxygen cycle cannot be disconnected from the carbon dioxide cycle; as oxygen rises it would be anticipated that carbon dioxide would fall. The carbon dioxide cycle is coupled with the climate, and this in turn affects the growth of both consumers and producers. The environmental feedback from carbon dioxide and climate would further stabilize the system. Once the initial oxygen crisis was over the Proterozoic could have been a comfortable time for Gaia, apart from the persistent annoyance of planetesimals. The natural level of carbon dioxide would have provided a pleasant climate, and no great effort would be needed to regulate it.
A bizarre consequence of the appearance of oxygen was the advent of the world's first nuclear reactors. Nuclear power from its inception has rarely been described publicly except in hyperbole. The impression has been given that to design and construct a nuclear reactor is a feat unique to physical science and engineering creativity. It is chastening to find that, in the Proterozoic, an unassertive community of modest bacteria built a set of nuclear reactors that ran for millions of years.
This extraordinary event occurred 1.8 eons ago at a place now called Oklo in Gabon, Africa, and was discovered quite by accident. At Oklo, there is a mine that supplies uranium mainly for the French nuclear industry. During the 1970s, a shipment of uranium from Oklo was found to be depleted in the fissionable isotope 235U. Natural uranium is always of the same isotopic composition -- 99.27 percent 238U, 0.72 percent of 235U, and traces of 234U. Only the 235U isotope can take part in the chain reactions necessary for power production or for explosions. Naturally, the fissionable isotope is guarded carefully and its proportion in uranium subjected to thorough and repeated scrutiny. Imagine the shock that must have passed through the French atomic energy agency when it was discovered that the shipment of uranium had a much smaller proportion of 235U than normal. Had some clandestine group in Africa or France found a way to extract the potent fissionable isotope, and were they now storing this for use in terrorist nuclear weapons? Had someone stolen the uranium ore from the mine and substituted spent uranium from a nuclear industry elsewhere? Whatever had happened, a sinister explanation seemed likely. The truth, when it came, was not only a fascinating piece of science but must also have been an immense relief to minds troubled with images of tons of undiluted 235U in the hands of fanatics.
The chemistry of the element uranium is such that it is insoluble in water under oxygen-free conditions, but readily soluble in water in the presence of oxygen. When enough oxygen appeared in the Proterozoic to render the ground water oxidizing, uranium in the rocks began to dissolve and, as the uranyl ion, became one of the many elements present in trace quantities in flowing streams. The strength of the uranium solution would have been at most no more than a few parts per million, and uranium would have been but one of many ions in solution. In the place that is now Oklo such a stream flowed into an algal mat that included microorganisms with a strange capacity to collect and concentrate uranium specifically. They performed their unconscious task so well that eventually enough uranium oxide was deposited in the pure state for a nuclear reaction to start.
When more than a "critical mass" of uranium containing the fissionable isotope is gathered together in one place there is a self-sustaining chain reaction. The fission of uranium atoms sets free neutrons that cause the fission of more uranium atoms and more neutrons and so on. Provided that the number of neutrons produced balances those that escape, or are absorbed by other atoms, the reactor continues. This kind of reactor is not explosive; indeed it is self-regulating. The presence of water, through its ability to slow and reflect neutrons, is an essential feature of the reactor. When the power output increases, water boils away and the nuclear reaction slows down. A nuclear fission reaction is a perverse kind of fire; it burns better when well watered. The Oklo reactors ran gently at the kilowatt-power level for millions of years and used up a fair amount of the natural 235U in doing so.
The presence of the Oklo reactors confirms an oxidizing environment. In the absence of oxygen, uranium is not water soluble. It is just as well that it is not; when life started 3.6 eons back, uranium was much more enriched in the fissile isotope 235U. This isotope decays more rapidly than the common isotope 238U, and at life's beginning the proportion of fissile uranium was not 0.7 percent as now but 33 percent. Uranium so enriched could have been the source of spectacular nuclear fireworks had any bacteria then been unwise enough to concentrate it. This also suggests that the atmosphere was not oxidizing in the early Archean.
Bacteria could not have debated the costs and benefits of nuclear power. The fact that the reactors ran so long and that there was more than one of them suggests that replenishment must have occurred and that the radiation and nuclear waste from the reactor was not a deterrent to that ancient bacterial ecosystem. (The distribution of stable fission products around the reactor site is also valuable evidence to suggest that the problems of nuclear waste disposal now are nowhere near so difficult or dangerous as the feverish pronouncements of the antinuclear movement would suggest.) The Oklo reactors are a splendid example of geophysiological homeostasis. They illustrate how specific minerals can be segregated and concentrated in the pure state -- an act of profound negentropy in itself, but also an invaluable subsystem of numerous geophysiological processes. The separation of silica by the diatoms and of calcium carbonate by coccolithophoridons and other living organisms, both in nearly pure form, are such processes and have had a profound effect on the evolution of the Earth.
If some descendant of the alien chemist who visited in the Archean returned in the Proterozoic, it would find an Earth not so different from now. The sky would have been a paler shade of blue with perhaps less cloud cover. On the beach, the sea would be blue-gray, rather than the brown of the Archean. Inland, behind the sand dunes and pebbles, the bacterial mats would lie, enlivened by the origin of certain green and golden yellow algae, protecting the anoxic sector that overlay and kept intact the evaporite beds beneath with their deep layers of lifeless salt, the accumulation of thousands or even millions of years. Out to sea there would again be the rock structures of stromatolite colonies. I wonder if our alien would have observed a remarkable geophysiological property of reefs that has recently been revealed in coral reefs. Satellite photographs showed that the wavelength of ocean waves in the vicinity of the reefs was unusual and unexpected for the surface wind and sea conditions. Subsequent investigation uncovered the remarkable fact that the coral microorganisms secreted a lipid substance that formed a monomolecular layer on the ocean surface and so altered its surface tension as to modify the waves. It is engaging to speculate about the geophysiological development of this remarkable action in microorganisms, and to wonder when it developed and whether it is a mechanism for protecting the reefs from wave damage.
During the Proterozoic, the constant rain of planetesimals continued. As well as numerous smaller ones, there were at least ten that did damage to Gaia comparable in severity to that of a burn affecting 60 percent of the skin area of a human. These events are not in themselves the main interest; we have no detailed information of the dates and consequences of their occurrence. What is interesting is the recovery of the system from these insults. We are tolerably certain that none of them killed Gaia, so that a new Gaia had to be born from the debris. The ability to recover from major perturbations is a test of the health of a geophysiological system; the fact that life persisted and recovered from so many of these catastrophes is more evidence in favor of the existence of a powerful homeostatic system on Earth.
At the beginning of the Proterozoic, the Sun was cooler. The problem facing Gaia was to keep the carbon dioxide greenhouse from collapsing and so causing the Earth to freeze. Without the cooling tendency of life today, the Earth would be uncomfortably hot. It could be said that life is at present keeping the Earth cool by pumping down carbon dioxide. In the middle ages of the Proterozoic, some 1.5 eons ago, the Sun's output was about just right for life and no great effort was needed for thermostasis. The atmospheric carbon dioxide was probably around one percent by volume. It was at a level where physicists and geophysiologists would have no cause for disagreement.