by G.S. Johal*, D.M. Huber1
Department of Botany & Plant Pathology, Purdue University, 915 West State Street, West Lafayette, IN 47907, USA
European Journal of Agronomy
Article history: Received 5 June 2008; Received in revised form 24 February 2009; Accepted 20 April 2009
© 2009 Elsevier B.V. All rights reserved.
NOTICE: THIS WORK MAY BE PROTECTED BY COPYRIGHT
YOU ARE REQUIRED TO READ THE COPYRIGHT NOTICE AT THIS LINK BEFORE YOU READ THE FOLLOWING WORK, THAT IS AVAILABLE SOLELY FOR PRIVATE STUDY, SCHOLARSHIP OR RESEARCH PURSUANT TO 17 U.S.C. SECTION 107 AND 108. IN THE EVENT THAT THE LIBRARY DETERMINES THAT UNLAWFUL COPYING OF THIS WORK HAS OCCURRED, THE LIBRARY HAS THE RIGHT TO BLOCK THE I.P. ADDRESS AT WHICH THE UNLAWFUL COPYING APPEARED TO HAVE OCCURRED. THANK YOU FOR RESPECTING THE RIGHTS OF COPYRIGHT OWNERS.
Keywords: Glyphosate; Plant defense; Disease; Manganese; Micronutrient; Transgenic; Soil microflora
ABSTRACT
Glyphosate, N-(phosphonomethyl)glycine, is the most extensively used herbicide in the history of agriculture. Weed management programs in glyphosate resistant (GR) field crops have provided highly effective weed control, simplified management decisions, and given cleaner harvested products. However, this relatively simple, broad-spectrum, systemic herbicide can have extensive unintended effects on nutrient efficiency and disease severity, thereby threatening its agricultural sustainability. A significant increase in disease severity associated with the wide spread application of the glyphosate herbicide can be the result of direct glyphosate-induced weakening of plant defenses and increased pathogen population and virulence. Indirect effects of glyphosate on disease predisposition result from immobilization of specific micronutrients involved in disease resistance, reduced growth and vigor of the plant from accumulation of glyphosate in meristematic root, shoot, and reproductive tissues, altered physiological efficiency, or modification of the soil-microflora affecting the availability of nutrients involved in physiological disease resistance. Strategies to ameliorate the predisposing effects of glyphosate on disease include judicious selection of herbicide application rates, micronutrient amendment, glyphosate detoxification in meristematic tissues and soil, changes in cultural practices to enhance micronutrient availability for plant uptake, and biological amendment with glyphosate-resistant-microbes for nitrogen fixation and nutrient availability. Given that recommended doses of glyphosate are often many times higher than needed to control weeds, we believe the most prudent method to reduce the detrimental effects of glyphosate on GR crops will be to use this herbicide in as small a dose as practically needed. Such a frugal approach will not only curtail disease predisposition of GR crops, but will also benefit the grower and the environment.
1. Introduction
Changes in agricultural practices such as crop rotation, crop sequence, tillage, and fertility that affect the soil-microflora or nutrient availability generally result in changes in disease expression (Datnoff et al., 2007; Englehard, 1989; Huber and Graham, 1999). This is commonly observed for soil-borne diseases where only limited innate resistance is available in commercial cultivars so that cultural controls become important management practices to minimize the impact of these diseases. Threatening to make things worse in this regard is the introduction of herbicide-resistant crops (canola, corn, cotton, soybeans, alfalfa, etc.) that are now grown extensively throughout the world. This new trend in agriculture has increased the usage and intensity of specific herbicides while limiting genetic diversity in the specific crops that have been genetically modified.
Herbicides are known to increase specific plant diseases (Altman and Campbell, 1977; Hornby et al., 1998; Mekwatanakarn and Sivasithamparam, 1987), and several are reported to influence micronutrient availability (Evans et al., 2007; Huber et al., 2004, 2005). Micronutrients are the activators or inhibitors of many critical physiological functions. Thus, a deficiency or change in availability of these regulatory elements can greatly affect plant growth and resistance to diseases and pests (Datnoff et al., 2007). The virulence mechanism of some pathogens such as Gaeumannomyces, Magnaporthe, Phymatotrichum, Corynespora, and Streptomyces involves Mn oxidation at the infection site to compromise the plant’s resistance mechanisms involving the shikimate pathway (Thompson and Huber, 2007). Isolates of these pathogens that cannot oxidize physiologically available Mn2+ to the nonavailable Mn4+ are a virulent and not able to cause significant tissue damage (Roseman et al., 1991). Production of the Mn oxidizing enzyme(s) occurs soon after spore germination and during epiphytic growth (Cheng, 2005; Schulze et al., 1995; Thompson et al., 2005). Environmental conditions that reduce the availability of micronutrients for plant uptake also predispose plants to disease (Huber and McCay-Buis, 1993;Huber and Graham, 1999;Thompson and Huber, 2007).
Table 1: Some diseases increased in glyphosate weed control programs.
Plant / Disease / Pathogen / References
Apple / Canker / Botryosphaeria dothidea / Rosenberger and Fargione (2004)
Banana / Panama disease / Fusarium oxysporum f. sp. cubense / Harper (2007)
Barley / Root rot / Magnaporthe grisea / Smiley et al. (1992)
Bean / Anthracnose / Colletotrichum lindemuthianum / Johal and Rahe (1984, 1988, 1990)
Bean / Damping off, root rot / Pythium spp. / Johal and Rahe (1984)
Bean / Root rot Fusarium solani f. sp. phaseoli / Harper (2007)
Bean / Hypocotyl rot / Phytophthora megasperma / Keen et al. (1982)
Canola / Crown rot / Fusarium spp. / Harper (2007)
Canola / Wilt / Fusarium oxysporum / Harper (2007), Large and McLaren (2002)
Citrus / Citrus variegated chlorosis / Xylella fastidiosa / Yamada (2006)
Citrus / Crown rot / Phytophthora spp. / Yamada (2006)
Cotton / Damping off / Pythium spp. / Harper (2007)
Cotton / Bunchy top / Manganese deficiency / Harper (2007)
Cotton / Wilt / F. oxysporum f. sp. vasinfectum / Harper (2007)
Grape / Black goo / Phaeomoniella chlamydospora / Harper (2007)
Melon / Root rot / Monosporascus cannonbalus / --
Soybeans / Root rot / Corynespora cassiicola / Huber et al. (2005)
Soybeans / Target spot / Corynespora cassiicola / Huber et al. (2005)
Soybeans / Sudden Death Syndrome / Fusarium solani f. sp. glycines / Keen et al. (1982)
Soybeans / Root rot / Phytophthora megasperma / Keen et al. (1982)
Soybeans / Cyst nematode / Heterodera glycines / Geisler et al. (2002), Kremer et al. (2000)
Soybeans / White mold / Sclerotinia sclerotiorum / Harper (2007)
Sugar beet / Yellows / Fusarium oxysporum f. sp. betae / Larson et al. (2006)
Sugar beet / Root rot / Rhizoctonia solani / Larson et al. (2006)
Sugarcane / Decline / Marasmius spp. / Huber (unpublished)
Tomato / Crown root rot / Fusarium / Bramhall and Higgins (1988)
Tomato / Wilt / Fusarium oxysporum f. sp. pisi / Harper (2007)
Various / Canker / Phytophthora spp. / Harper (2007)
Weeds / Biocontrol / Myrothecium verrucaria / Boyette et al. (2006)
Wheat / Bare patch / Rhizoctonia solani / Harper (2007)
Wheat / Glume blotch / Septoria spp. / Harper (2007)
Wheat / Root rot / Fusarium spp./ Fernandez et al. (2005, 2007), Harper (2007)
Wheat / Head scab / Fusarium graminearum / Fernandez et al. (2005)
Wheat / Take-all / Gaeumannomyces graminis / Hornby et al. (1998)
The herbicide glyphosate, N-(phosphonomethyl)glycine, is a strong systemic metal chelator and was initially patented for that purpose (Bromilowet al., 1993). Its herbicidal action is by chelating with Mn, a cofactor for the 5-enolpyruvylshikimate-3-phosphate (EPSP) synthase enzyme in the shikimate pathway, to inhibit this metabolic pathway of plants and many microorganisms (Cerdeira and Duke, 2006; Grossbard and Atkinson, 1985; Jaworski, 1972). Many cations chelate with glyphosate, thus reducing its herbicidal efficacy (Bernards et al., 2005; Hickman et al., 2002). Plants with a compromised shikimatemetabolism are predisposed to various plant pathogens (Johal and Rahe, 1988; Rahe et al., 1990), and glyphosate is patented as a synergist for mycoherbicides to enhance the virulence and pathogenicity of organisms used for biological weed control (Boyette et al., 2006; Duke and Cerdeira, 2005). The synergistic activity of glyphosate weed control in predisposing plants to infectious organisms has been observed for many diseases (Table 1), and the extensive use of glyphosate in agriculture is a significant factor in the increased severity or “reemergence” of diseases once considered efficiently managed.
The extensive adoption of Roundup Ready® crops such as soybeans, canola, cotton, and corn has intensified the application of glyphosate in these production systems. The applied glyphosate is readily translocated to roots and released throughout the rhizosphere in root exudates of Roundup Ready® plants as well as glyphosate-sensitive plants (Bromilow et al., 1993; Grossbard and Atkinson, 1985). The toxicmicrobial effects of glyphosate are cumulative with continued use so that Mn deficiency is now observed in areas that were previously considered Mn sufficient because of reduced populations of Mn-reducing soil organisms (Huber, unpublished). The presence of the glyphosate-resistance gene in corn and soybeans also reduces Mn uptake and physiological efficiency (Dodds et al., 2002a,b,c; Gordon, 2006; Reichenberger, 2007). Along with glyphosate-induced Mn deficiency, there has been a gradual recognition of increased disease severity (Harper, 2007; Larson et al., 2006). A few examples are presented to illustrate this relationship.
2. Some diseases increased by glyphosate
2.1. Corynespora root rot of soybean
The damage from Corynespora root rot, previously considered minor, may become economically damaging in Roundup Ready® soybeans since application of glyphosate to Roundup Ready® soybeans greatly increases severity of this disease (Fig. 1). This fungal root rot is more severe when glyphosate is applied to soybeans under weedy conditions even though the weeds may not be hosts for Corynespora cassiicola. The weeds serve to translocate and release more glyphosate into the rhizosphere environment to reduce the population of Mn-reducing organisms and increase Mnoxidizing organisms. This change in soil biology limits manganese availability for plant uptake and active defense reactions, and acts synergistically with Corynespora to increase disease (Huber et al., 2005).
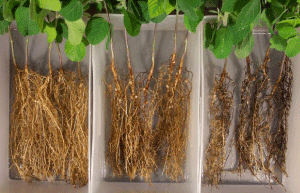
Fig. 1. Increased severity of Corynespora root rot after glyphosate application to RoundupReady® soybeans. Non-inoculated control (left), inoculated plants (center), inoculated plants sprayed with glyphosate (right).
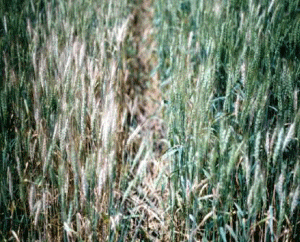
Fig. 2. More severe take-all root rot of wheat grown following Roundup Ready® soybeans sprayed with glyphosate (left) than following Roundup Ready® soybeans grown with a non-glyphosate herbicide (right).
Table 2
Some conditions affecting the form of nitrogen, manganese availability, and severity of take-all, rice blast, potato scab, Phymatotrichum root rot, and corn stalk rot (after Thompson and Huber, 2007).
Soil factor or cultural practice / Favored N form (NH4 vs. NO3) / Manganese availability / Severity of these diseases
Low soil pH / NH4 / Increase / Decrease
Green manures (some) / NH4 / Increase / Decrease
Ammonium fertilizers / NH4 / Increase / Decrease
Irrigation (some) / NH4 / Increase / Decrease
Firm seed bed / NH4 / Increase / Decrease
Nitrification inhibitors / NH4 / Increase / Decrease
Soil fumigation / NH4 / Increase / Decrease
Metal sulfides / NH4 / Increase / Decrease
High soil pH / NO3 / Decrease / Increase
Lime / NO3 / Decrease / Increase
Nitrate fertilizers / NO3 / Decrease / Increase
Manure / NO3 / Decrease / Increase
Low soil moisture / NO3 / Decrease / Increase
Loose seed bed / NO3 / Decrease / Increase
2.2. Take-all of cereal crops
The most comprehensive understanding of the interaction of micronutrients influenced by glyphosate and disease is with the take-all disease of cereals. Increased take-all of cereals after a preplant “burn-down” use of glyphosate has been recognized for over 15 years (Hornby et al., 1998). Take-all is also increased when glyphosate is applied to Roundup Ready® soybeans the preceding year compared with the use of a non-glyphosate herbicide (Fig. 2). All of the conditions known to affect Mn availability are inversely related to the severity of take-all (and other diseases, Table 2) so that those conditions that increase the availability of Mn for plant uptake generally reduce take-all, and those that reduce Mn availability increase take-all (Huber and McCay-Buis, 1993). Microorganisms proposed for biological control of this disease such as Bacillus cereus and Trichoderma konigii are all strong Mn reducers that increase Mn availability in the rhizosphere (Huber and McCay-Buis, 1993; McCay-Buis, 1998; Rengel et al., 1996). In contrast, the addition of Mn-oxidizing organisms increases take-all (Crowley and Rengel, 1999; McCay-Buis, 1998; Rengel, 1999; Thompson et al., 1998). Gaeumannomyces graminis is a strong Mn oxidizer in soil and as it grows externally along plant roots (Thompson et al., 2000, 2005). Isolates of Gaeumannomyces that cannot oxidize Mn are avirulent, and isolates that oxidize Mn only at certain temperatures are virulent only at temperatures where they can oxidize Mn (Roseman et al., 1991).
Species of Gramineae such as rye (Secale cereale L.) that are efficient in Mn uptake are resistant to take-all compared with the relatively inefficient, highly susceptible wheat (Triticum aestivum L.) (Hornby et al., 1998). In contrast, resistance of oats to take-all is associated with glycocyanide root exudates that are toxic toMnoxidizing organisms in the rhizosphere.Oats, as a precrop for wheat, provide effective control of take-all in many areas because of the induced shift in soil biological activity that is less favorable for Mn oxidation. The biological activity favoring Mn availability reduces take-all severity for two or more subsequent wheat crops even though there is little change in the pathogen population (Huber and McCay-Buis, 1993). Glyphosate, in contrast to oats, is toxic to Mn-reducing and N-fixing organisms in soil so that the availability of nitrogen andMnin soilmay bemarkedly compromised (Huber et al., 2004). Low levels of residual glyphosate in soil also reduce root uptake and translocation of Fe,Mn, and Cu (Eker et al., 2006; Ozturk et al., 2008). Increased take-all root, crown, and foot rot of cereals following glyphosate applications (Hornby et al., 1998; Huber and McCay-Buis, 1993) may be the result of reduced resistance frominducedMn deficiency, inhibited root growth fromglyphosate accumulation in root tips, modified virulence of the pathogen, or an increase in synergistic Mn-oxidizing organisms in the rhizosphere.
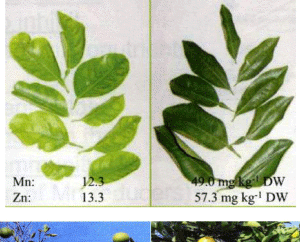
Fig. 3. Expression of citrus variegated chlorosis under glyphosate (left) compared with an alternative mulch (right) weed control program. All trees are infected with the CVC pathogen, Xylella fastidiosa (after Yamada, 2006). Left: severe Mn and Zn deficiency, and eventual severe decline in vigor with the glyphosate weed management program. Right: restoration of tissue nutrient levels and productivity of X. fastidiosa infected trees under the non-glyphosate mulch system.
2.3. Diseases caused by Xylella fastidiosa
Various diseases caused byX. fastidiosa are referred to as “emerging” or “reemerging” diseases as glyphosate weed management programs for their respective crops have intensified. These diseases (Pierce’s disease of grapevine, plum scorch, almond scorch, citrus variegated chlorosis, coffee blight, citrus blight, alfalfa dwarf, pecan decline, etc.) are characterized by a loss of vigor, slow decline, micronutrient deficiency, and reduced productivity. The pathogen is an endophytic bacterium that colonizes xylem tissues and restricts nutrient translocation when plants are stressed. Citrus variegated chlorosis (CVC) was first described on oranges in Brazil in 1987 and is also recognized in Puerto Rico. An early symptom of this disease is a variegated chlorosis of foliage (Fig. 3) similar to a deficiency of, and associated with, a drop in tissue levels of Mn and Zn (Li et al., 1996). Normal flushes of new growth are sparse or absent, fruit is small, “skirts” of trees move up, and trees enter a serious decline in growth and productivity. A similar disease referred to as “citrus blight” occurs worldwide and causes the death of several hundred thousand citrus trees annually in the United States (Derrick and Timmer, 2000; Timmer, 2000). Yamada (2006) developed the only known control for CVC, and properly managed trees return to full productivity even though the pathogen may still be present. Control of CVC emphasizes elimination of glyphosate and adoption of an alternative grass mulchweed control program for citrus orchards in Brazil (Yamada and Castro, 2005). This control strategy uses optimally fertilized Brachiaria grass grown between the tree rows. The grass is mowed twice a year to provide a 10–15cm mulch under the citrus trees for weed control and nutrition. Natural mineralization of this mulch inhibits nitrification to provide an ammonium source of nutrition for the citrus trees, Mn and Zn tissue levels are restored to sufficiency levels, and trees in early to mid-decline produce a new flush of growth. Full productivity is restored within a few years. Removing glyphosate from the citrus production system also has significantly reduced the occurrence of Phytophthora crown rot.
2.4. Fusarium diseases
Various diseases caused by Fusarium spp. are increased by glyphosate (Fernandez et al., 2005; Sanogo et al., 2000, 2001). Glyphosate has made crops susceptible to normally non-pathogenic isolates of Fusarium, and the population of Fusarium increases in soil after glyphosate application (Levesque et al., 1987; Kremer et al., 2000). Glyphosate predisposes tomato to Fusarium crown and root rot by inhibiting the plant’s structural and defense barriers (Bramhall and Higgins, 1988). Cotton growers in Australia and the Western United States have seen a resurgence of Fusarium wilt since the introduction of Roundup Ready® cotton, and previously high levels of wilt resistance appear to be less effective under glyphosate management programs (Harper, 2007). Glyphosate also breaks resistance to cyst nematodes in soybeans (Geisler et al., 2002). The increased Fusarium yellows and Rhizoctonia solani diseases of Roundup Ready® sugar beets prompted Larson et al. (2006) to comment that “precautions need to be taken when certain soil-borne diseases are present if weed management for sugar beet is to include post-emergence glyphosate treatments.” These authors also reported that the sugar beet variety resistant to Rhizoctonia was as susceptible to this pathogen as the susceptible variety after glyphosate application regardless of the time of inoculation.
Fusarium head scab of cereals and other diseases caused by Fusarium spp. increase following glyphosate applications (Fernandez et al., 2005; Larson et al., 2006), and previously established “cardinal” conditions (precipitation, flowering, and temperatures above 26 "C) for head scab are modified when glyphosate is applied prior to a susceptible cereal crop (Fernandez et al., 2005, 2007). Glyphosate modifies plant nitrogen metabolism similar to high temperature-induced changes that provide susceptibility to head scab (Huber, unpublished) so that head scab and the mycotoxins produced by the causal fungi are now prevalent in cooler areas where they were rarely observed before the extensive use of glyphosate (Fernandez et al., 2005, 2007). Similar changes in nitrogen and carbohydrate metabolism provide transient resistance of wheat and soybeans to rust after glyphosate application (Anderson and Kolmer, 2005; Feng et al., 2005, 2007).
The Palouse area of Washington, Idaho, and Oregon in the United States has had a long history of pea, lentil, and wheat production on the deep loess soils characteristic of the area; however, pea and lentil yields have been in slow decline as symbiotic nitrogen fixation is reduced and Fusarium diseases increased commensurate with the extensive use of glyphosate for no-till wheat production. Pea and lentil production are now uneconomical in some areas, and production is rapidly moving from the Palouse to Montana where glyphosate usage has been more limited. The loss of legumes in crop rotations in the Palouse area can result in serious degradation of these once highly productive soils with few economical, alternative crops available as replacements. A newFusariumwilt of canola caused by F. oxysporum and F. avenaceum has caused severe yield reductions in nutrient poor soils of Alberta and Saskatchewan, Canada since 2000, but has not yet becomea problem in the Mn-rich soils in the Red River valley (Lange and McLaren, 2002).
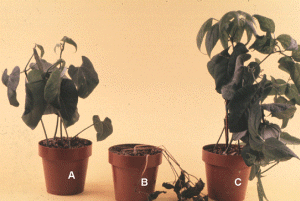
Fig. 4. Fate of glyphosate-treated (10!g plant−1) bean plants grown in (A) vermiculite and (B) field soil 20 days after glyphosate treatment, and (C) non-glyphosate treated control plants. Glyphosate treated plants in field soil (B) collapsed 10 days after glyphosate treatment from Pythium infection.
3. Predisposition to disease underlies the herbicidal efficacy of glyphosate
Inhibition of EPSP synthase initially was considered to be the sole target of glyphosate in plants. It was believed that this mode of action would kill plants by starving them of aromatic amino acids through deregulation of the shikimate pathway (Cerdeira and Duke, 2006; Grossbard and Atkinson, 1985; Jaworski, 1972). This, however, did not explain some aspects of the death caused by glyphosate. For instance, glyphosate must be translocated to roots to be effective, although growth of the plant stops soon after application of the herbicide. In addition, effects of sublethal doses of this herbicide on perennial plants sometimes appear a year after exposure and persist for two or more years (Rahe et al., 1990). These characteristics of glyphosate-induced injury suggest that the herbicidal action of glyphosatewas more than simply the starvation of treated plants of aromatic amino acids as assumed initially (Rahe et al., 1990).
Intrigued by these observations and the possibility that something about the root environment may contribute to the herbicidal action of glyphosate, a systematic research effort was launched in the early 1980s that led to the following findings (Levesque and Rahe, 1992; Rahe et al., 1990):
(1) The herbicidal efficacy of glyphosate is largely due to colonization of roots of affected plants by soil-borne pathogens (Fig. 4) (Johal and Rahe, 1984).
(2) Two pathogens that are most important in this regard are Pythium, an oomycete, and Fusarium, an ascomycete. Both of these pathogens are ubiquitous in agricultural and other soils.
(3) Plants growing in sterile medium do not die even though their growth is temporarily inhibited by glyphosate.
(4) Amending sterile media with Pythium or Fusarium restores the ability of glyphosate to kill plants.
(5) Both Pythium and Fusarium begin to colonize plants within a day or two of glyphosate application to foliar parts of the plant (Fig. 4) (Johal and Rahe, 1984; Levesque et al., 1993).
(6) The amount of glyphosate needed to kill plants in natural soils is much lower than the recommended dose.
These results suggested that glyphosate was somehow compromising the ability of plants to defend against rhizosphere inhabiting pathogens.
4. Mechanisms of predisposition to disease
Plants rely on multiple components of defense to deter pathogens following infection (Hammond-Kosack and Jones, 2000). Many of these active resistance components are derived from the phenylpropanoid pathway, which acquires almost all of its precursors (notably phenylalanine and chorismate) fromthe shikimic acid pathway (Hammond-Kosack and Jones, 2000; Dixon et al., 2002). A key inducible defense component associated with the shikimic acid pathway is the production of antimicrobial phytoalexins that accumulate rapidly at the site of infection. Lignification of cellwalls at and around the infection site is another shikimate-derived component that functions to fortify cells and ensure isolation of the pathogen at the infection site. Production of salicylic acid (SA) following infection represents another component of inducible defense. SA is synthesized either directly from chorismic acid or indirectly through phenylalanine. Although SA is not antimicrobial per se, it functions to signal and coordinate various defenses following challenge by a pathogen; however, its direct role in plant–pathogen interactions involving root tissue remains unclear. Another defense component that relies on three final products of the shikimic acid pathway – tryptophan, tyrosine and phenylalanine – is the production of a diverse variety of pathogenesis-related (PR) proteins that function to curtail the advance of a pathogen. Many kinds of PR proteins have been identified (Hammond-Kosack and Jones, 2000).
Given the reliance of many plant defenses on the shikimic acid pathway, and the fact that glyphosate blocks this pathway, it is not surprising that this herbicide would render plants more susceptible to pathogens. Keen et al. (1982) were the first to show that by inhibiting the phytoalexin glyceollin, glyphosate was able to compromise resistance of soybeans to Phytophthora megasperma f. sp. glycines. Using the bean-anthracnose pathosystem, Johal and Rahe (1988, 1990) demonstrated that, while glyphosate did not interfere with the hypersensitive reaction (HR) of incompatible interactions, it suppressed significantly the production of all four of the bean phytoalexins. As a result, the pathogen was able to kill the plant if it escaped the localized HR, a situation that occurred only with glyphosate-treated plants (Fig. 5). The effect of glyphosate on the compatible bean anthracnose interaction was even more dramatic (Johal and Rahe, 1990). Glyphosate almost completely suppressed the production of phytoalexins associated with susceptible lesion containment and permitted the pathogen to invade unimpededly until the entire hypocotyl collapsed (Figs. 6 and 7). As little as 2% of the recommended herbicidal rate of glyphosate was enough to transform normally delimited lesions typical of anthracnose into constantly expanding lesions (Johal and Rahe, 1990).
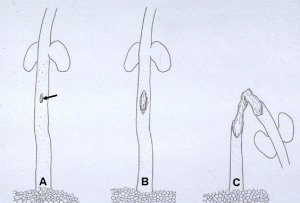
Fig. 5. Diagramatic representation of glyphosate-treated bean seedlings following inoculation with an incompatible race of Colletotrichum lindemuthianum. (A) Dots all over the hypocotyl represent hypersensitive reaction (HR) sites (cells) incited by the pathogen on spray inoculation. Arrow indicates the site where a drop of glyphosate (10!g) was placed. (B) The fungus normally contained inside HR cells sometimes escapes near the glyphosate treatment site and results in a susceptible lesion (arrow). (C) The lesion continues to expand to kill the plant after glyphosate treatment.
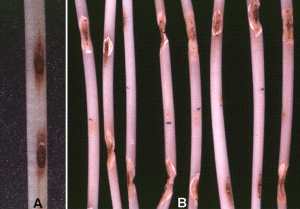
Fig. 6. Anthracnose lesions on bean hypocotyls in the (A) absence of glyphosate and (B) presence of glyphosate. A 10!g drop of glyphosate (black dot) was placed near the center of the hypocotyls one day after inoculation with C. lindemuthianum in (B). Note the loss of lesion delimitation and collapse of tissue seven days after glyphosate treatment.
The defense studies mentioned above were confined largely to diseases of aerial parts of host plants. There are indications that defense components may vary significantly in root tissue that are in intimate and continuous contact with potential pathogens (Hammond-Kosack and Jones, 2000). For instance, roots do not rely on HR-mediated defense to contend with pathogens, although the exact defense components that keep roots pathogen-free are only partially understood. To gain an insight into what contributes to glyphosate-induced susceptibility of French beans (Phaseolus vulgaris) to Pythium, Liu et al. (1995, 1997) assessed phytoalexins as well as lignification of root tissue in response to glyphosate treatment. By comparing phytoalexins in roots of bean seedlings grown in different media, they concluded that phytoalexins were induced by soil microorganisms. Interestingly, while phytoalexin accumulation was affected only modestly by glyphosate in response to exposure to Pythium, lignification (a process requiring Mn) was suppressed significantly. Thus, enhanced colonization by Pythium in roots of bean seedlings treated with foliar applied glyphosate occurs as a result of glyphosate interference with lignin-based defense mechanisms (Liu et al., 1997). However, these results also suggest that sustained production of phytoalexins in response to Pythium infection is maintained temporarily following glyphosate treatment, whereas lignification is not.
5. Roundup Ready® plants and disease predisposition
Given that the herbicidal activity of glyphosate is mediated largely by its ability to lower plant immunity to pathogens, the status of Roundup Ready® plants with regard to such predisposition following glyphosate treatment becomes a serious consideration. For reasons that were not explained, Cerdeira and Duke (2006) contended that reduced resistance to pathogens in response to glyphosate treatment should not occur in Roundup Ready® plants. This is a misconception that can hold true only if the Roundup Ready® transgene following glyphosate treatment operates and behaves in exactly the same manner as the native EPSP synthase gene does in the absence of glyphosate. Such a scenario is possible only if the Roundup Ready® transgene is completely insensitive to glyphosate and is also as efficacious as the native EPSP synthase gene is in the absence of glyphosate. In addition, the Roundup Ready® gene has to match exactly the transcriptional activity of the native gene in every tissue of the plant and under all conditions, both normal and stressful. This is a tall order of requirements that is unlikely to be fulfilled by the present day Roundup Ready® transgenics, thus making it highly probable that our Roundup Ready® crops are vulnerable to glyphosate toxicity under at least some conditions. One such condition could arise when the level of glyphosate exceeds the ability of the transgenic enzyme to tolerate it, and yet another may develop if the transgene fails to match the transcriptional activity and profile of the native gene under conditions of biotic stress. Both of these scenarios are possible and, if they develop, it is very likely they would enhance the vulnerability of Roundup Ready® plants to fungal diseases following Roundup application.
Glyphosate treatment of transgenic crops to manage weeds can also promote disease damage indirectly by impacting the inoculum potential of pathogens. Shortly after soilborne fungi’s causative role was revealed in the herbicidal efficacy of glyphosate (Johal and Rahe, 1984), Levesque et al. (1987) documented a significant, albeit temporary, spike in the level of fungal pathogens in the rhizosphere following glyphosate application to weeds. This prompted the speculation that such a buildup of pathogen load could have ill effects for subsequent crop plants. This, indeed, was found to be the case in barley fields in which significant yield reductions were witnessed if the crop was planted within a few days after glyphosate application (Smiley et al., 1992). Although the latter study was conducted on non Roundup Ready® barley, it is likely that a similar boost in the inoculum potential of pathogens in the rhizosphere (also called ‘green bridge’) could lead to enhanced root rot problems in Roundup Ready® crops as well.
A prudent way to avoid disease enhancement is to decrease the concentration of glyphosate applied to Roundup Ready® crops. Many studies have documented that the levels of glyphosate necessary to kill or compromise the health of many weeds are several fold lower than the generally recommended application rates (Rahe et al., 1990). An alternative to using insensitive EPSP synthase genes to generate glyphosate-resistant plants might be to use genes that degrade glyphosate. Three such genes which inactivate glyphosate by oxidation (the Gox gene), acetylation (the Gat gene) or decarboxylation (the Gdc gene) have become available in recent years (Cerdeira and Duke, 2006; Dill, 2005). If the problem persists, there is also the possibility of stacking a resistant EPSP synthase gene with a glyphosate metabolism gene as has been done in canola (Dill, 2005).Amajor disadvantage of this strategy is that it may encourage the application of higher levels of glyphosate than needed. In turn, this would not only impact the environment negatively but also would hasten the evolution of resistant weeds and thereby further threaten sustainability of this herbicide.
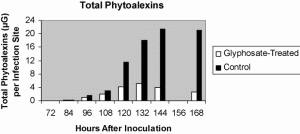
Fig. 7. Glyphosate suppression of phytoalexins in compatible bean anthracnose lesions by 10!g glyphosate (after Johal and Rahe, 1990).
6. Strategies to ameliorate glyphosate predisposition to disease
Several strategies may be deployed to reduce glyphosate induced predisposition to disease. These strategies primarily focus on four aspects of the glyphosate-disease-environment interaction, i.e.:
(1) minimizing non-target exposure to glyphosate by limiting the rates of glyphosate used,
(2) enhancing micronutrient sufficiency to maintain optimum plant physiological function and resistance,
(3) detoxifying accumulated glyphosate in root tips and other meristematic tissues to restore growth potential, and
(4) moderating glyphosate toxicity to rhizosphere microbes or restoring critical microbial components damaged by glyphosate released in root exudates.
6.1. Minimizing non-target exposure to glyphosate by limiting the rates of glyphosate used
As stated earlier, the rates of glyphosate generally recommended for herbicide use are far in excess of the amount required to kill most weeds. Excess application has occurred primarily as a result of advertising promotions, ease of application, increasing weed resistance, low cost of the product, and apathy towards the extensive non-target environmental effects of glyphosate. Very low levels of residual glyphosate in soil can greatly impede the availability and uptake of Mn, Fe, Cu, and Zn with subsequent translocation to vegetative tissues also impeded (Eker et al., 2006; Ozturk et al., 2008). This limitation in uptake and translocation can greatly impede the “replenishing” of these critical micronutrients and restoration of physiological resistance mechanisms dependent on them after nutrient immobilization in tissues by the applied glyphosate. A more judicious use of glyphosate would appear essential to maintain sustainable crop production efficiency.
6.2. Enhancing micronutrient sufficiency to maintain optimum plant physiological function and resistance
Most micronutrients are readily absorbed after foliar application, a common method of fertilization; however, some micronutrients, such as Mn, are relatively immobile and are not basipitally translocated to roots where soil-borne root, hypocotyl, crown and vascular pathogens are established (Marschner, 1995; Thompson and Huber, 2007). Thus, although foliar application of Mn can provide nutrient sufficiency to foliar tissues for this essential element, it would not be effective in detoxifying accumulated glyphosate in root tip meristematic tissues or maintaining physiological resistance dependent on the shikimate pathway in root tissues because it is relatively immobile in the plant and does not move downward in the phloem.
A combination of foliar applied Mn with more mobile elements such as Cu or Zn could be more effective in detoxifying glyphosate in root tissues than Mn alone. Difficulties in meeting plant needs for Mn are further compounded since soil-applied Mn can be readily oxidized by soil organisms to the Mn4+ form that is not available for plant uptake (Marschner, 1995; Thompson and Huber, 2007). Reduced physiological efficiency of Roundup Ready® crops (Dodds et al., 2002a,b,c; Gordon, 2006; Zobiole et al., 2009) require higher levels of Mn to achieve nutrient sufficiency and comparable productivity as their non-genetically modified isolines (Reichenberger, 2007). Rates of Mn applied to Roundup Ready® soybeans required for comparable yield with non-RR soybean approached toxicity when applied to the isogenic non-Roundup Ready® soybean (Gordon, 2006; Reichenberger, 2007). The simultaneous application of many nutrients with glyphosate (“tank mixes”) results in their immobilization and non-availability for plant physiological functions. Full physiological efficiency from nutrient application may not be achieved unless the micronutrients are applied eight to fifteen days after the glyphosate is applied. This is necessary to prevent chelation and immobilization by residual glyphosate in tissues that renders them physiologically unavailable (Huber et al., 2004; Severson, 2006), although earlier applications may be more effective in detoxifying tissue-bound glyphosate.
6.3. Detoxifying accumulated glyphosate in meristematic tissues
Reduced root growth from the accumulation of glyphosate in root tips results in less contact of the roots with dispersed nutrients in the soil profile and may negate tolerance of plants to soilborne pathogens based on their ability to “outgrow” the damage caused from loss of root tissue. Likewise, glyphosate accumulates in active meristematic tissue in shoots and developing fruits to inhibit growth of these tissues. Calcium, Mg, and micronutrients that chelate with glyphosate can reduce its biological activity and restore some of the potential physiological activity in these tissues. These “detoxifying” elements can come from within the plant or from further uptake from the soil. Thus, it is important to maintain mineral sufficiency in plant tissues and their ready availability in soil for plant uptake. This may be achieved by soil or foliar applied nutrients (Bernards et al., 2005; Huber et al., 2004; Reichenberger, 2007) if other environmental restraints are considered.
6.4. Eliminating glyphosate toxicity to rhizosphere microbes or restoring critical microbial components damaged by glyphosate released in root exudates
Detoxifying glyphosate in root exudates may occur in highly calcareous soils or soils with high levels of soluble metal nutrients through chelation to reduce its impact on soil organisms. Toxicity of glyphosate to Mn-reducing and synergistic nitrogen-fixing organisms in the rhizosphere can have serious consequences for sustainability of legume production. Regular inoculation of legume crops with synergistic nitrogen-fixing organisms may be required in many areas for maximal productivity where extended applications of glyphosate have eliminated them from the soil profile. Development of glyphosate-tolerant nitrogen-fixing and Mn-reducing organisms would be beneficial in many of these situations, and especially for perennial Roundup Ready® legume crops such as alfalfa.
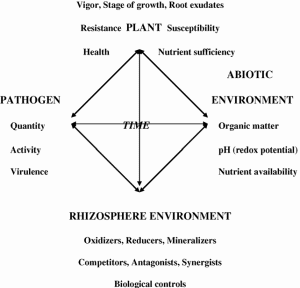
Fig. 8. The four primary interacting factors influencing nutrient availability and disease that are affected by glyphosate.
7. Summary
Extended use of glyphosate can significantly increase the severity of various diseases by impacting all four of the interacting components of the “plant disease diamond” comprised of the plant, abiotic and biotic environments, and pathogen (Fig. 8). Reduced growth, impaired defenses, impaired uptake and translocation of nutrients, and altered physiology of plants by glyphosate can affect susceptibility or tolerance to various diseases. Glyphosate chelation of nutrients in the plant and soil can render those nutrients immobile and unavailable for plant use or uptake, while toxicity to essential synergistic and beneficial soil organisms (Purcell, 2001) further reduces availability of nutrients that are critical for a plant’s physiological defense to disease. Glyphosate stimulation of fungal growth and enhanced virulence of pathogens such as Fusarium, Gaeumannomyces, Phytophthora, Pythium, and Xylella can have serious consequences for sustainable production of a wide range of susceptible crops and lead to the functional loss of genetic resistance that is dependent on metabolites through the shikimate pathway (Larson et al., 2006). Nutrient balance is important because each element functions as part of a delicately balanced, interdependent physiological system with the plant’s genetics and the environment. Maximal utilization of cultural and management practices that increase the availability of nutrients (Table 2) to negate the deleterious effects of glyphosate should be incorporated into crop production programs to facilitate optimal production efficiency and sustainable disease control. It is important to understand the effect of glyphosate on the chemical and biological properties of soils and its overall effects on the agricultural production system to permit its judicious use. Ignoring potential non-target detrimental side effects of any chemical, especially used as heavily as glyphosate, may have dire consequences for agriculture such as rendering soils infertile, crops non-productive, and plants less nutritious (Altman and Campbell, 1977). To do otherwise might well compromise not only agricultural sustainability, but also the health and well-being of animals and humans (Ozturk et al., 2008).
Acknowledgements
We are grateful to Dr. Larry Dunkle for providing a critical review of the manuscript, and to Lynn Johal for editorial comments.
References
Altman, J., Campbell, C.L., 1977. Effect of herbicides on plant diseases. Annu. Rev. Phytopathol. 15, 361–385.
Anderson, J.A.,Kolmer, J.A., 2005. Rust control in glyphosate tolerant wheat following the application of the herbicide glyphosate. Plant Dis. 88, 1136–1142.
Bernards, M.L., Thelen, K.D., Muthukumaran, R.J., McCracken, J.L., 2005. Glyphosate interaction with manganese in tank mixtures and its effect on glyphosate absorption and translocation.Weed Sci. 53, 787–794.
Boyette, C.D., Reddy, K.N., Hoagland, R.E., 2006. Glyphosate and bioherbicide interaction for controlling kudzu (Pueraria lobata), redvine (Brunnichia ovata), and trumpetcreeper (Campsis radicans). Biocontrol Sci. Tech. 16, 1067–1077.
Bramhall, R.A., Higgins, V.J., 1988. The effect of glyphosate on resistance of tomato to Fusarium crown and root rot disease and on the formation of host structural defensive barriers. Can. J. Bot. 66, 1547–1555.
Bromilow, R.H., Chamberlain, K., Tench, A.J.,Williams, R.H., 1993. Phloem translocation of strong acids – glyphosate, substituted phosphonic, and sulfonic acids – in Ricinus communis L. Pestic. Sci. 37, 39–47.
Cerdeira, A.L., Duke, S.O., 2006. The current status and environmental impacts of glyphosate-resistant crops: a review. J. Environ. Qual. 35, 1633–1658.
Cheng, M.-W., 2005. Manganese transition states during infection and early pathogenesis in rice blast. M.S. Thesis. Purdue University, West Lafayette, Ind.
Crowley, D.E., Rengel, Z., 1999. Biology and chemistry of nutrient availability in the rhizosphere. In: Rengel, Z. (Ed.), Mineral Nutrition of Crops: Fundamental Mechanisms and Implications. Food Products Press, London, pp. 1–40 (Chapter 1).
Datnoff, L.E., Elmer, W.H., Huber, D.M., 2007. Mineral Nutrition and Plant Disease. APS Press, St. Paul, MN.
Derrick, K.S., Timmer, L.W., 2000. Citrus blight and other diseases of recalcitrant etiology. Annu. Rev. Phytopathol. 38, 181–205.
Dill, G., 2005. Glyphosate resistant crops: history, status and future. Pest Manag. Sci. 61, 219–224.
Dixon, R.A., Achnine, L., Kota, P., Liu, C.-J., Reddy, M.S., Wang, L., 2002. The phenylpropanoid pathway and plant defense—a genomics perspective. Mol. Plant Pathol. 3, 371–390.
Dodds, D.M., Hickman, M.V., Huber, D.M., 2002a. Micronutrient uptake by isogenic glyphosate tolerant and normal corn. Proc. Proc.Weed Sci. Soc. Am. 42, 2.
Dodds, D.M., Huber, D.M., Hickman, M.V., 2002b. Micronutrient levels in normal and glyphosate-resistant soybeans. Proc. NC-Weed Sci. Soc. Am. 57, 107.
Dodds, D.M., Huber, D.M., Hickman, M.V., Shaw, D.R., 2002c. Hybrid and glyphosate application effects on nutrient uptake in corn. Proc.Weed Sci. Soc. Am. 43, 4.
Duke, S.O., Cerdeira, L., 2005. Potential environmental impacts of herbicide-resistant crops. Collect. Biosaf. Rev. 2, 66–143.
Eker, S., Ozturk, L., Yazici, A., Erenoglu, B., Romheld, V., Cakmak, I., 2006. Foliar applied glyphosate substantially reduced uptake and transport of iron and manganese in sunflower (Helianthus annuus L.) plants. J. Agric. Food Chem. 54, 10019–10025.
Englehard, A.W. (Ed.), 1989. Management of Diseases with Macro and Microelements. American Phytopathological Society, St. Paul, MN.
Evans, I.R., Solberg, E., Huber, D.M., 2007. Copper and plant disease. In: Datnoff, L.E., Elmer,W.H., Huber, D.M. (Eds.), Mineral Nutrition and Plant Disease. APS Press, St. Paul, MN, pp. 177–188 (Chapter 12).
Feng, P.C.C., Baley, J., Clinton, W.P., Bunkers, G.J., Alibhai, M.F., Paulitz, T.C., Kidwell, K.K., 2005. Glyphosate inhibits rust diseases in glyphosate-resistant wheat and soybean. Proc. Natl. Acad. Sci. U.S.A. 102, 17290–17295.
Feng, P.C.C., Clark, C., Andrade, G.C., Baibl, M.C., Caldwell, P., 2007. The control of Asian rust by glyphosate in glyphosate-resistant soybeans. Pest Manag. Sci. 63, 1526.
Fernandez, M.R., Selles, F., Gehl, D., DePauw, R.M., Zentner, R.P., 2005. Crop production factors associated with Fusarium head blight in spring wheat in eastern Saskatchewan. Crop Sci. 45, 1908–1916.
Fernandez, M.R., Zentner, R.P., DePauw, R.M., Gehl, D.T., Stevenson, F.C., 2007. Impacts of crop production factors on Fusarium head blight in barley in eastern Saskatchewan. Crop Sci. 47, 1574–1584.
Geisler, L., Graef, G., Wilson, J., Schimelfenig, J., 2002. Interaction of glyphosate tolerance with soybean cyst nematode resistance. Phytopathology 92, S529.
Gordon, B., 2006. Manganese nutrition of glyphosate-resistant and conventional soybeans. Better Crops 91, 12–13.
Grossbard, E., Atkinson, D., 1985. The Herbicide Glyphosate. Butterworths, London.
Hammond-Kosack, K., Jones, J.D.G., 2000. Responses to plant pathogens. In: Buchanan, B.B., Gruissem, W., Jones, R.L. (Eds.), Biochemistry and Molecular Biology of Plants. ASPP, Rockville, MD, pp. 1102–1156.
Harper, M., 2007. The Review of the Moratorium on GM Canola. Australia. http://www.dpi.vic.gov.au/dpi/nrenfa.ns ... 8881F86CA- 2572E300074EEF89E6C67B4668BD2A7CA256FB70001BAB8.
Hickman, M.V., Dodds, D.M., Huber, D.M., 2002. Micronutrient interactions reduce glyphosate efficacy on tall fescue. Proc.Weed Sci. Soc. Am. 42, 18.
Hornby, D., Bateman, G.L., Gutteridge, R.J., Lucas, P., Osbourn, A.E.,Ward, E., Yarham, D.J., 1998. Take-all Disease of Cereals: A Regional Perspective. CAB International, Wallingford, UK.
Huber, D.M., McCay-Buis, T.S., 1993. A multiple component analysis of the take-all disease of cereals. Plant Dis. 77, 437–447.
Huber, D.M., Graham, R.D., 1999. The role of nutrition in crop resistance and tolerance to diseases. In: Rengel, Z. (Ed.), Mineral Nutrition of Crops: Fundamental Mechanisms and Implications. Food Products Press, London, pp. 169–204.
Huber, D.M., Leuck, J.D., Smith,W.C., Christmas, E.P., 2004. Induced manganese deficiency in GM soybeans. In: Northcentral Fert. Extension Conf., Des Moines, IA, November 2004.
Huber, D.M., Cheng,M.W.,Winsor, B.A., 2005. Association of severe Corynespora root rot of soybean with glyphosate-killed giant ragweed. Phytopathology 95, S45.
Jaworski, E.G., 1972. Mode of action of N-phosphonomethyl-glycine: inhibition of aromatic amino acid biosynthesis. J. Agric. Food Chem. 20, 1195–1198.
Johal, G.S., Rahe, J.E., 1984. Effect of soilborne plant-pathogenic fungi on the herbicidal action of glyphosate on bean seedlings. Phytopathology 74, 950–955.
Johal, G.S., Rahe, J.E., 1988. Glyphosate, hypersensitivity and phytoalexin accumulation in the incompatible bean anthracnose host–parasite interaction. Physiol. Mol. Plant Pathol. 32, 267–281.
Johal, G.S., Rahe, J.E., 1990. Role of phytoalexins in the suppression of resistance of Phaseolus vulgaris to Colletotrichum lindemuthianum by glyphosate. Can. J. Plant Pathol. 12, 225–235.
Keen, N.T., Holliday, M.J., Yoshikawa, M., 1982. Effects of glyphosate on glyceollin production and the expression of resistance to Phytophthora megasperma f. sp. glycinea in soybean. Phytopathology 72, 1467–1470.
Kremer, R.J., Donald, P.A., Keaster, A.J., Minor, H.C., 2000. Herbicide impact on Fusarium spp. and soybean cyst nematode in glyphosate-tolerant soybean. Agron. Abstr., p257.
Lange, R., McLaren, D., 2002. Fusarium Wilt—A New Disease of Canola. http://www.umanitoba.ca/afs/agronomists conf/2002/pdf/lange.pdf.
Larson, R.L., Hill,A.L., Fenwick,A., Kniss,A.R., Hanson, L.E., Miller, S.D., 2006. Influence of glyphosate on Rhizoctonia and Fusarium root rot in sugar beet. Pest Manag. Sci. 62, 182–192.
Levesque, C.A., Rahe, J.E., et al., 1987. Effects of glyphosate on Fusarium spp.: its influence on root colonization of weeds, propagule density in the soil, and on crop emergence. Can. J. Microbiol. 33, 354–360.
Levesque, C.A., Rahe, J.E., 1992. Herbicide interactions with fungal root pathogens, with special reference to glyphosate. Annu. Rev. Phytopathol. 30, 579–602. 152 G.S. Johal, D.M. Huber / Europ. J. Agronomy 31 (2009) 144–152
Levesque, C.A., Rahe, J.E., Eaves,D.M., 1993. Fungal colonization of glyphosate treated seedlings using a new root plating technique. Mycol. Res. 97, 299–306.
Li, W.B., Donadio, L.C., Sempionato, O.R., Miranda, V.S., 1996. Resistance or tolerance of citrus species and cultivars to citrus variegated chlorosis. Proc. Int. Soc. Citricult. 1, 216–218.
Liu, L., Punja, Z.K., Rahe, J.E., 1995. Effect of Pythium spp. and glyphosate on phytoalexin production and exudation by bean (Phaseolus vulgaris L.) roots grown in different media. Physiol. Mol. Plant Pathol. 47, 391–405.
Liu, L., Punja, Z.K., Rahe, J.E., 1997. Altered root exudation and suppression of induced lignification a smechanisms of predisposition by glyphosate of beanroots (Phaseolus vulgaris L.) to colonization by Pythium spp. Physiol. Mol. Plant Pathol. 51, 111–127.
Marschner, H., 1995. Mineral Nutrition of Higher Plants. In: second ed. Academic Press, London, 889 pp.
McCay-Buis, T.S., 1998. Ramifications of microbial interactions conditioning take-all of wheat. Ph.D. Thesis. Purdue University,West Lafayette, Indiana.
Mekwatanakarn, P., Sivasithamparam, K., 1987. Effect of certain herbicides on soil microbial populations and their influence on saprophytic growth in soil and pathogenicity of the take-all fungus. Biol. Fertil. Soils 5, 175–180.
Ozturk, L., Yazici, A., Eker, S., Gokmen, O., Romheld, V., Cakmak, I., 2008. Glyphosate inhibition of ferric reductase activity in iron deficient sunflower roots. New Phytol. 177, 899–906.
Purcell, L.C., 2001. Physiological determinants of soybean yield limitations. USDACRIS Accession No.: 0164131; project No.:ARK01559,Univ. Arkansas, Fayetteville.
Rahe, J.E., Levesque, C.A., Johal, G.S., 1990. Synergistic role of soil fungi in the herbicidal efficacy of glyphosate. In: Hoagland, R.E. (Ed.), Biological Weed Control Using Microbes and Microbial Products as Herbicides. Symposium, April 9–14, 1989. American Chemical Society, Washington, DC, pp. 260–275.
Reichenberger, L., 2007. Missing micronutrients: using glyphosate is complicating the uptake of some minor nutrients. In: The Furrow, pp. 22–23.
Rengel, Z. (Ed.), 1999. Mineral Nutrition of Crops: Fundamental Mechanisms and Implications. Food Products Press, London.
Rengel, Z., Gutteridge, R., Hirsch, P., Hornby, D., 1996. Plant genotype, micronutrient fertilization and take-all infection influence bacterial populations in the rhizosphere of wheat. Plant Soil 183, 269–277.
Roseman, T.S., Graham, R.D., Arnott, H.J., Huber, D.M., 1991. The interaction of temperature with virulence and manganese oxidizing potential in the epidemiology of Gaeumannomyces graminis. Phytopathology 81, S1215.
Rosenberger, D., Fargione, M., 2004. Apple Pest Report 12, 6. University of Maine Cooperative Extension Service.
Sanogo, S., Yang, X.B., Scherm, H., 2000. Effects of herbicides on Fusarium solani f. sp. glycines and development of sudden death syndrome in glyphosate-tolerant soybean. Phytopathology 90, 57–66.
Sanogo, S., Yang, X.B., Lundeen, P., 2001. Field response of glyphosate-tolerant soybean to herbicides and sudden death syndrome. Plant Dis. 85, 773–779.
Schulze, D.G., McCay-Buis, T.S., Sutton, S.R., Huber, D.M., 1995. Manganese oxidation states in Gaeumannomyces-infested wheat rhizospheres probedby micro-XANES spectroscopy. Phytopathology 85, 990–994.
Severson, R., 2006. Influence of Roundup Herbicide on Manganese Nutrition of Soybean. http://www.nwroc.umn.edu/Cropping Issues/NW Crop trials/2005/Sybn glyph + manganese.pdf.
Smiley, R.W., Ogg, A.G., Cook, R.J., 1992. Influence of glyphosate on Rhizoctonia root rot, growth, and yield of barley. Plant Dis. 76, 937–942.
Thompson, I.A., Huber, D.M., 2007. Manganese and plant disease. In: Datnoff, L.E., Elmer,W.H., Huber, D.M. (Eds.), Mineral Nutrition and Plant Disease. APS Press, St. Paul, MN, pp. 139–153 (Chapter 10).
Thompson, I.A., Guest, C.A., Schulze, D.G., Huber, D.M., 1998. Manganese reduction and uptake in wheat rhizospheres as influenced by manganese reducing and oxidizing bacteria. Phytopathology 88, S118.
Thompson, I.A., Huber, D.M., Schulze, D.G., 2000. In situ oxidation and accumulation of manganese by the causal agent of the take-all disease on wheat (Gaeumannomyces graminis). Phytopathology 90, S77.
Thompson, I.A., Huber, D.M., Guest, C.A., Sculze, D.G., 2005. Fungal manganese oxidation in a reduced soil. Environ. Microbiol. 7, 1480–1487.
Timmer, L.W., 2000. Blight. In: Compendium of Citrus Diseases. APS Press, St. Paul, MN, pp. 66–67.
Yamada, T., 2006. Informac¸ ões Agronomicas 116. IPNI-International Plant Nutrition Institute, Piracicaba-SP, Brazil. http://www.ipni.org.br/ppiweb/pbrazil.nsf/ $webindex/article=93F35608032570B5004E419AD0187284!opendocument.
Yamada, T., Castro, P.R., 2005. Glifosato, herbicida com singular modo de ac¸ ão: Efeitos secundários e implicac¸ ões fisiológicas e agronômicas. http://www.ipni. org.br/ppiweb/pbrazil.nsf/$webcontentsbydate!OpenView&Start=1&Count=60 &Expand=1.1#1.1.
Zobiole, L.H.S., deOliveira, R.S., Huber, D.M., Constantin, J., Castro, C, deOliveira, F.A., de Oliveira, A., 2009. Glyphosate reduces shoot concentrations of mineral nutrients in glyphosate-resistant soybeans. Plant Soil (In Press).
________________
Notes:
* Corresponding author. Tel.: +1 765 494 4448; fax: +1 765 494 0363.
E-mail addresses: [email protected] (G.S. Johal), [email protected] (D.M. Huber).
1 Tel.: +1 765 714 6825.