by U.S. Global Change Research Program. Authors: David M. Anderson, Donald F. Boesch, Virginia R. Burkett, Lynne M. Carter, Stewart J. Cohen, Nancy B. Grimm, Jerry L. Hatfield, Katharine Hayhoe, Anthony C. Janetos, Jack A. Kaye, Jay H. Lawrimore, James J. McCarthy, A. David McGuire, Edward L. Miles, Evan Mills, Jonathan T. Overpeck, Jonathan A. Patz, Roger S. Pulwarty, Benjamin D. Santer, Michael J. Savonis, H. Gerry Schwartz, Jr., Eileen L. Shea, John M.R. Stone, Bradley H. Udall, John E. Walsh, Michael F. Wehner, Thomas J. Wilbanks, Donald J. Wuebbles
June, 2009
NOTICE: THIS WORK MAY BE PROTECTED BY COPYRIGHT
YOU ARE REQUIRED TO READ THE COPYRIGHT NOTICE AT THIS LINK BEFORE YOU READ THE FOLLOWING WORK, THAT IS AVAILABLE SOLELY FOR PRIVATE STUDY, SCHOLARSHIP OR RESEARCH PURSUANT TO 17 U.S.C. SECTION 107 AND 108. IN THE EVENT THAT THE LIBRARY DETERMINES THAT UNLAWFUL COPYING OF THIS WORK HAS OCCURRED, THE LIBRARY HAS THE RIGHT TO BLOCK THE I.P. ADDRESS AT WHICH THE UNLAWFUL COPYING APPEARED TO HAVE OCCURRED. THANK YOU FOR RESPECTING THE RIGHTS OF COPYRIGHT OWNERS.
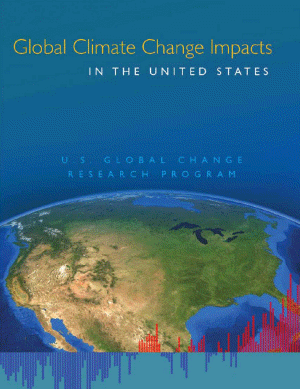
Table of Contents:
• Opening Pages
• About this Report
• Executive Summary
• Global Climate Change
• National Climate Change
• Climate Change Impacts by Sector
o Water Resources
o Energy Supply and Use
o Transportation
o Agriculture
o Ecosystems
o Human Health
o Society
• Regional Climate Change Impacts
o Northeast
o Southeast
o Midwest
o Great Plains
o Southwest
o Northwest
o Alaska
o Islands
o Coasts
• An Agenda for Climate Impacts Science
• Concluding Thoughts
• Author Team Biographies
• Primary Sources of Information
• Acronyms and Abbreviations
• References
• Photo and Figure Credits
The projected rapid rate and large amount of climate change over this century will challenge the ability of society and natural systems to adapt.
***
800,000 Year Record of Carbon Dioxide Concentration
Analysis of air bubbles trapped in an Antarctic ice core extending back 800,000 years documents the Earth’s changing carbon dioxide concentration. Over this long period, natural factors have caused the atmospheric carbon dioxide concentration to vary within a range of about 170 to 300 parts per million (ppm). Temperature-related data make clear that these variations have played a central role in determining the global climate. As a result of human activities, the present carbon dioxide concentration of about 385 ppm is about 30 percent above its highest level over at least the last 800,000 years. In the absence of strong control measures, emissions projected for this century would result in the carbon dioxide concentration increasing to a level that is roughly 2 to 3 times the highest level occurring over the glacial-interglacial era that spans the last 800,000 or more years.
***
***
Global emissions of carbon dioxide have been accelerating. The growth rate increased from 1.3 percent per year in the 1990s to 3.3 percent per year between 2000 and 2006. The increasing emissions of carbon dioxide are the primary cause of the increased concentration of carbon dioxide observed in the atmosphere. There is also evidence that a smaller fraction of the annual human-induced emissions is now being taken up than in the past, leading to a greater fraction remaining in the atmosphere and an accelerating rate of increase in the carbon dioxide concentration.
***
As the carbon dioxide concentration in the air increases, more carbon dioxide is absorbed into the world’s oceans, leading to their acidification. This makes less calcium carbonate available for corals and other sea life to build their skeletons and shells. If carbon dioxide concentrations continue to rise and the resulting acidification proceeds, eventually, corals and other ocean life that rely on calcium carbonate will not be able to build these skeletons and shells at all. ... Under projections for the future, it is very unlikely that calcium carbonate saturation levels will be adequate to support coral reefs in any U.S. waters.
***
The warming trend that is apparent in all of these temperature records is confirmed by other independent observations, such as the melting of Arctic sea ice, the retreat of mountain glaciers on every continent, reductions in the extent of snow cover, earlier blooming of plants in spring, and increased melting of the Greenland and Antarctic ice sheets. Because snow and ice reflect the Sun’s heat, this melting causes more heat to be absorbed, which causes more melting, resulting in another feedback loop.
***
After at least 2,000 years of little change, sea level rose by roughly 8 inches over the past century. Satellite data available over the past 15 years show sea level rising at a rate roughly double the rate observed over the past century.
***
The Earth has major ice sheets on Greenland and Antarctica. These ice sheets are currently losing ice volume by increased melting and calving of icebergs, contributing to sea-level rise. The Greenland Ice Sheet has also been experiencing record amounts of surface melting, and a large increase in the rate of mass loss in the past decade. If the entire Greenland Ice Sheet melted, it would raise sea level by about 20 feet. The Antarctic Ice Sheet consists of two portions, the West Antarctic Ice Sheet and the East Antarctic Ice Sheet. The West Antarctic Ice Sheet, the more vulnerable to melting of the two, contains enough water to raise global sea levels by about 16 to 20 feet. If the East Antarctic Ice Sheet melted entirely, it would raise global sea level by about 200 feet. Complete melting of these ice sheets over this century or the next is thought to be virtually impossible, although past climate records provide precedent for very significant decreases in ice volume, and therefore increases in sea level.
***
The observed changes in some climate variables, such as Arctic sea ice, some aspects of precipitation, and patterns of surface pressure, appear to be proceeding much more rapidly than models have projected. The reasons for these differences are not well understood.
***
Rapid ice sheet collapse with related sea-level rise is another type of abrupt change that is not well understood or modeled and that poses a risk for the future. Recent observations show that melting on the surface of an ice sheet produces water that flows down through large cracks that create conduits through the ice to the base of the ice sheet where it lubricates ice previously frozen to the rock below. Further, the interaction with warm ocean water, where ice meets the sea, can lead to sudden losses in ice mass and accompanying rapid global sea-level rise. Observations indicate that ice loss has increased dramatically over the last decade, though scientists are not yet confident that they can project how the ice sheets will respond in the future.
There are also concerns regarding the potential for abrupt release of methane from thawing of frozen soils, from the sea floor, and from wetlands in the tropics and the Arctic. While analyses suggest that an abrupt release of methane is very unlikely to occur within 100 years, it is very likely that warming will accelerate the pace of chronic methane emissions from these sources, potentially increasing the rate of global temperature rise.
A third major area of concern regarding possible abrupt change involves the operation of the ocean currents that transport vast quantities of heat around the globe. One branch of the ocean circulation is in the North Atlantic. In this region, warm water flows northward from the tropics to the North Atlantic in the upper layer of the ocean, while cold water flows back from the North Atlantic to the tropics in the ocean’s deep layers, creating a “conveyor belt” for heat. Changes in this circulation have profound impacts on the global climate system, from changes in African and Indian monsoon rainfall, to atmospheric circulation relevant to hurricanes, to changes in climate over North America and Western Europe.
Recent findings indicate that it is very likely that the strength of this North Atlantic circulation will decrease over the course of this century in response to increasing greenhouse gases. This is expected because warming increases the melting of glaciers and ice sheets and the resulting runoff of freshwater to the sea. This additional water is virtually salt-free, which makes it less dense than sea water. Increased precipitation also contributes fresh, less-dense water to the ocean. As a result, less surface water is dense enough to sink, thereby reducing the conveyor belt’s transport of heat. The best estimate is that the strength of this circulation will decrease 25 to 30 percent in this century, leading to a reduction in heat transfer to the North Atlantic. It is considered very unlikely that this circulation would collapse entirely during the next 100 years or so, though it cannot be ruled out. While very unlikely, the potential consequences of such an abrupt event would be severe.
***
When human influences are removed from the model experiments, results suggest that the surface of the Earth would actually have cooled slightly over the last 50 years.
***
The IPCC emission scenarios also do not encompass the full range of possible futures: emissions can change less than those scenarios imply, or they can change more. Recent carbon dioxide emissions are, in fact, above the highest emissions scenario developed by the IPCC. Whether this will continue is uncertain.
***
By the end of the century, the average U.S. temperature is projected to increase by approximately 7 to 11°F under the higher emissions scenario and by approximately 4 to 6.5°F under the lower emissions scenario. ... A variety of research studies suggest that a further 2°F increase (relative to the 1980-1999 period) would lead to severe, widespread, and irreversible impacts.
***
Scenarios that stabilize carbon dioxide below 450 ppm offer an increased chance of avoiding dangerous climate change.
***
Generally, higher latitudes are projected to receive more precipitation, while the dry belt that lies just outside the tropics expands further poleward, and also receives less rain. Increases in tropical precipitation are projected during rainy seasons (such as monsoons), and especially over the tropical Pacific. Certain regions, including the U.S. West (especially the Southwest) and the Mediterranean, are expected to become drier. The widespread trend toward more heavy downpours is expected to continue, with precipitation becoming less frequent but more intense. More precipitation is expected to fall as rain rather than snow.
***
Global Increase in Heavy Precipitation 1900 to 2100
***
If greenhouse gas emissions continue to increase, by the 2040s more than half of European summers will be hotter than the summer of 2003, and by the end of this century, a summer as hot as that of 2003 will be considered unusually cool.
***
There is also the possibility of even larger changes in climate than current scenarios and models project. Not all changes in the climate are gradual. The long record of climate found in ice cores, tree rings, and other natural records show that Earth’s climate patterns have undergone rapid shifts from one stable state to another within as short a period as a decade. The occurrence of abrupt changes in climate becomes increasingly likely as the human disturbance of the climate system grows. Such changes can occur so rapidly that they would challenge the ability of human and natural systems to adapt.|
***
U.S. average temperature has risen more than 2ºF over the past 50 years ... National temperatures vary much more than global temperatures, in part because of the moderating influence of the oceans on global temperatures.
***
Over the past 30 years, temperatures have risen faster in winter than in any other season, with average winter temperatures in the Midwest and northern Great Plains increasing more than 7ºF. ... On a seasonal basis, most of the United States is projected to experience greater warming in summer than in winter, [???] while Alaska experiences far more warming in winter than summer.
***
The number of days with high temperatures above 90°F is projected to increase throughout the country. Parts of the South that currently have about 60 days per year with temperatures over 90°F are projected to experience 150 or more days a year above 90°F by the end of this century, under a higher emissions scenario.
***
Sea ice is a very important part of the climate system. In addition to direct impacts on coastal areas of Alaska, it more broadly affects surface reflectivity, ocean currents, cloudiness, humidity, and the exchange of heat and moisture at the ocean’s surface. Open ocean water is darker in color than sea ice, which causes it to absorb more of the Sun’s heat, which increases the warming of the water even more. ... Arctic sea ice extent has fallen at a rate of 3 to 4 percent per decade over the last three decades. End-of-summer Arctic sea ice has fallen at an even faster rate of more than 11 percent per decade in that time. The observed decline in Arctic sea ice has been more rapid than projected by climate models. ... clear linkages between rising greenhouse gas concentrations and declines in Arctic sea ice have been identified in the climate record as far back as the early 1990s. The extreme loss in Arctic sea ice that occurred in 2007 would not have been possible without the long-term reductions that have coincided with a sustained increase in the atmospheric concentration of carbon dioxide and the rapid rise in global temperatures that have occurred since the mid-1970s. The total volume of Arctic sea ice in 2008 was likely a record low because the ice was unusually thin.
***
Since the industrial revolution, the United States has been the world’s largest emitter of heat-trapping gases. With 4.5 percent of world's population, the United States is responsible for about 28 percent of the human-induced heat-trapping gases in the atmosphere today.
***
Fires release carbon dioxide, so years with many large fires result in more carbon release and less uptake as natural sinks (the vegetation) are lost. Similarly, the trees destroyed by intense storms or droughts release carbon dioxide as they decompose, and the loss results in reduced strength of natural sinks until regrowth is well underway. For example, Hurricane Katrina killed or severely damaged over 320 million large trees. As these trees decompose over the next few years, they will release an amount of carbon dioxide equivalent to that taken up by all U.S. forests in a year.
***
Increased air temperatures lead to higher water temperatures, which have already been detected in many streams, especially during low-flow periods. In lakes and reservoirs, higher water temperatures lead to longer periods of summer stratification (when surface and bottom waters do not mix). Dissolved oxygen is reduced in lakes, reservoirs, and rivers at higher temperatures. Oxygen is an essential resource for many living things, and its availability is reduced at higher temperatures both because the amount that can be dissolved in water is lower and because respiration rates of living things are higher. Low oxygen stresses aquatic animals such as coldwater fish and the insects and crustaceans on which they feed. Lower oxygen levels also decrease the self-purification capabilities of rivers.
The negative effects of water pollution, including sediments, nitrogen from agriculture, disease pathogens, pesticides, herbicides, salt, and thermal pollution, will be amplified by observed and projected increases in precipitation intensity and longer periods when streamflows are low. The U.S. Environmental Protection Agency expects the number of waterways considered “impaired” by water pollution to increase. Heavy downpours lead to increased sediment in runoff and outbreaks of waterborne diseases. Increases in pollution carried to lakes, estuaries, and the coastal ocean, especially when coupled with increased temperature, can result in blooms of harmful algae and bacteria.
***
Sea-level rise is expected to increase saltwater intrusion into coastal freshwater aquifers, making some unusable without desalination. Increased evaporation or reduced recharge into coastal aquifers exacerbates saltwater intrusion. ... Desalination requires large amounts of energy to produce freshwater.
***
The interface between streams and groundwater is an important site for pollution removal by microorganisms. Their activity will change in response to increased temperature and increased or decreased streamflow as climate changes, and this will affect water quality. Like water quality, research on the impacts of climate change on groundwater has been minimal.
***
The nation’s drinking water and wastewater infrastructure is aging. In older cities, some buried water mains are over 100 years old and breaks of these lines are a significant problem. Sewer overflows resulting in the discharge of untreated wastewater also occur frequently. Heavier downpours will exacerbate existing problems in many cities, especially where storm-water catchments and sewers are combined.
***
Higher temperatures and longer dry periods are expected to lead to increased water demand for irrigation. ... Higher temperatures are projected to increase cooling water withdrawals by electrical generating stations. In addition, greater cooling requirements in summer will increase electricity use, which in turn will require more cooling water for power plants. ... in addition to cooling, air conditioners also remove moisture from the air; thus the increase in humidity projected to accompany global warming is likely to increase electricity consumption by air conditioners even further. ...The demand for cooling energy increases from 5 to 20 percent per 1.8°F of warming.
... Withdrawals of freshwater used to cool power plants that use heat to generate electricity are very large, nearly equaling the water withdrawn for irrigation. Water consumption by power plants is about 20 percent of all non-agricultural uses, or half that of all domestic use.
In the water sector, two very unusual attributes of water, significant weight due to its relatively high density, and high heat capacity, make water use energy intensive. Large amounts of energy are needed for pumping, heating, and treating drinking water and wastewater. Water supply and treatment consumes roughly 4 percent of the nation’s power supply, and electricity accounts for about 75 percent of the cost of municipal water processing and transport. In California, 30 percent of all non-power plant natural gas is used for water-related activities.
***
The ability to modify operational rules and water allocations is likely to be critical for the protection of infrastructure, for public safety, to ensure reliability of water delivery, and to protect the environment. There are, however, many institutional and legal barriers to such changes in both the short and long term. ... [For example], conserving water does not necessarily lead to a right to that saved water, thus creating a disincentive for conservation.
***
U.S. energy supply is dominated by fossil fuels. Petroleum, the top source of energy shown above, is primarily used for transportation (70 percent of oil use).
***
An estimated 60,000 miles of coastal highway are already exposed to periodic flooding from coastal storms and high waves. Some of these highways currently serve as evacuation routes during hurricanes and other coastal storms, and these routes could become seriously compromised in the future.
***
The loss of coastal wetlands and barrier islands will lead to further coastal erosion due to the loss of natural protection from wave action.
***
Regional Spotlight: New York Metropolitan Area: With the potential for significant sea-level rise estimated under continued high levels of emissions, the combined effects of sea-level rise and storm surge are projected to increase the frequency of flooding. What is currently called a 100-year storm is projected to occur as often as every 10 years by late this century. Portions of lower Manhattan and coastal areas of Brooklyn, Queens, Staten Island, and Nassau County, would experience a marked increase in flooding frequency. Much of the critical transportation infrastructure, including tunnels, subways, and airports, lies well within the range of projected storm surge and would be flooded during such events.
***
Heavy downpours have already increased substantially in the United States; the heaviest 1 percent of precipitation events increased by 20 percent, while total precipitation increased by only 7 percent over the past century. Such intense precipitation is likely to increase the frequency and severity of events such as the Great Flood of 1993, which caused catastrophic flooding along 500 miles of the Mississippi and Missouri river system, paralyzing surface transportation systems, including rail, truck, and marine traffic. Major east-west traffic was halted for roughly six weeks in an area stretching from St. Louis, Missouri, west to Kansas City, Missouri and north to Chicago, Illinois, affecting one-quarter of all U.S. freight, which either originated or terminated in the flood-affected region.
***
Pipelines are likely to be damaged because intense precipitation can cause the ground to sink underneath the pipeline; in shallow river-beds, pipelines are more exposed to the elements and can be subject to scouring and shifting due to heavy precipitation.
***
Changes in silt and debris buildup resulting from extreme precipitation events will affect channel depth, increasing dredging costs.
***
Longer periods of extreme heat in summer can damage roads in several ways, including softening of asphalt that leads to rutting from heavy traffic. Sustained air temperature over 90°F is a significant threshold for such problems. Extreme heat can cause deformities in rail tracks, at minimum resulting in speed restrictions and, at worst, causing derailments. Extreme heat also causes thermal expansion of bridge joints, adversely affecting bridge operations and increasing maintenance costs. ... Increases in very hot days and heat waves are expected to limit construction activities due to health and safety concerns for highway workers.
***
Earlier spring snowmelt leads to increased number of forest fires. ... Wildfires are projected to increase, especially in the Southwest. ... There is also increased susceptibility to mudslides in areas deforested by wildfires.
***
If low water levels become more common because of drier conditions due to climate change, this could create problems for river traffic, reminiscent of the stranding of more than 4,000 barges on the Mississippi River during the drought in 1988. Freight movements in the region could be seriously impaired, and extensive dredging could be required to keep shipping channels open.
***
Extreme heat also affects aircraft lift; because hotter air is less dense, it reduces the lift produced by the wing and the thrust produced by the engine – problems exacerbated at high altitudes and high temperatures. As a result, planes need to take off faster, and if runways are not sufficiently long for aircraft to build up enough speed to generate lift, aircraft weight must be reduced. Thus, increases in extreme heat will result in payload restrictions, could cause flight cancellations and service disruptions at affected airports, and could require some airports to lengthen runways.
***
There will be a greater probability of infrastructure failures such as highway and rail bridge decks being displaced and railroad tracks being washed away. Storms leave debris on roads and rail lines, which can damage the infrastructure and interrupt travel and shipments of goods. In Louisiana, the Department of Transportation and Development spent $74 million for debris removal alone in the wake of hurricanes Katrina and Rita. The Mississippi Department of Transportation expected to spend in excess of $1 billion to replace the Biloxi and Bay St. Louis bridges, repair other portions of roadway, and remove debris.
***
Over the past 50 years, Alaska has warmed at more than twice the rate of the rest of the United States’ average. Its annual average temperature has increased 3.4°F, while winters have warmed even more, by 6.3°F.501 As a result, climate change impacts are much more pronounced than in other regions of the United States.
***
The grain-filling period (the time when the seed grows and matures) of wheat and other small grains shortens dramatically with rising temperatures. Analysis of crop responses suggests that even moderate increases in temperature will decrease yields of corn, wheat, sorghum, bean, rice, cotton, and peanut crops.
Some crops are particularly sensitive to high nighttime temperatures, which have been rising even faster than daytime temperatures. Nighttime temperatures are expected to continue to rise in the future. These changes in temperature are especially critical to the reproductive phase of growth because warm nights increase the respiration rate and reduce the amount of carbon that is captured during the day by photosynthesis to be retained in the fruit or grain. Further, as temperatures continue to rise and drought periods increase, crops will be more frequently exposed to temperature thresholds at which pollination and grain-set processes begin to fail and quality of vegetable crops decreases. Grain, soybean, and canola crops have relatively low optimal temperatures, and thus will have reduced yields and will increasingly begin to experience failure as warming proceeds. Common snap beans show substantial yield reduction when nighttime temperatures exceed 80°F.
Higher temperatures will mean a longer growing season for crops that do well in the heat, such as melon, okra, and sweet potato, but a shorter growing season for crops more suited to cooler conditions, such as potato, lettuce, broccoli, and spinach. Higher temperatures also cause plants to use more water to keep cool. But fruits, vegetables, and grains can suffer even under well-watered conditions if temperatures exceed the maximum level for pollen viability in a particular plant; if temperatures exceed the threshold for that plant, it won’t produce seed and so it won’t reproduce.
***
***
Fruits that require long winter chilling periods will experience declines. Many varieties of fruits (such as popular varieties of apples and berries) require between 400 and 1,800 cumulative hours below 45°F each winter to produce abundant yields the following summer and fall. By late this century, under higher emissions scenarios, winter temperatures in many important fruit-producing regions such as the Northeast will be too consistently warm to meet these requirements. Cranberries have a particularly high chilling requirement, and there are no known low-chill varieties. Massachusetts and New Jersey supply nearly half the nation’s cranberry crop. By the middle of this century, under higher emissions scenarios, it is unlikely that these areas will support cranberry production due to a lack of the winter chilling they need.
***
Ground-level ozone (a component of smog) is an air pollutant that is formed when nitrogen oxides emitted from fossil fuel burning interact with other compounds, such as unburned gasoline vapors, in the atmosphere, in the presence of sunlight. Higher air temperatures result in greater concentrations of ozone. Ozone levels at the land surface have risen in rural areas of the United States over the past 50 years, and they are forecast to continue increasing with warming, especially under higher emissions scenarios. Plants are sensitive to ozone, and crop yields are reduced as ozone levels increase. Some crops that are particularly sensitive to ozone pollution include soybeans, wheat, oats, green beans, peppers, and some types of cotton.
***
Mild winters and warm, early springs, which are beginning to occur more frequently as climate warms, induce premature plant development and blooming, resulting in exposure of vulnerable young plants and plant tissues to subsequent late-season frosts. ... reduced snow cover leaves young plants unprotected from spring frosts.
***
Storms with heavy rainfall often are accompanied by wind gusts, and both strong winds and rain can flatten crops, causing significant damage. Vegetable and fruit crops are sensitive to even short-term, minor stresses, and as such are particularly vulnerable to weather extremes.
***
Crop diseases in general are likely to increase as earlier springs and warmer winters allow proliferation and higher survival rates of disease pathogens and parasites.
***
Poison ivy thrives in air with extra carbon dioxide in it, growing bigger and producing a more toxic form of the oil, urushiol, which causes painful skin reactions in 80 percent of people.
***
Farmers are likely to respond to more aggressive and invasive weeds, insects, and pathogens with increased use of herbicides, insecticides, and fungicides. Where increases in water and chemical inputs become necessary, this will increase costs for the farmer, as well as having society-wide impacts by depleting water supply, increasing reactive nitrogen and pesticide loads to the environment, and increasing risks to food safety and human exposure to pesticides.|
***
On shortgrass prairie, a carbon dioxide enrichment experiment reduced the protein concentration of autumn forage below critical maintenance levels for livestock in 3 out of 4 years and reduced the digestibility of forage by 14 percent in mid-summer and by 10 percent in autumn.
***
Temperature and humidity interact to cause stress in animals, just as in humans; the higher the heat and humidity, the greater the stress and discomfort, and the larger the reduction in the animals’ ability to produce milk, gain weight, and reproduce. ... Nighttime recovery is an essential element of survival when livestock are stressed by extreme heat. A feature of recent heat waves is the lack of nighttime relief. Large numbers of deaths have occurred in recent heat waves, with individual states reporting losses of 5,000 head of cattle in a single heat wave in one summer. ... Heat stress reduces animals’ ability to cope with other stresses, such as diseases and parasites.
***
Butterfly Range Shifts Northward: ... Because their change in range is slow, most species are not expected to be able to keep up with the rapid climate change projected in the coming decades. ... A study of Edith’s checkerspot butterfly showed that 40 percent of the populations below 2,400 feet have gone extinct, despite the availability of otherwise suitable habitat and food supply. ... For butterflies, birds, and other species, one of the concerns with such changes in geographic range and timing of migration is the potential for mismatches between species and the resources they need to survive.
***
Forest tree species also are expected to shift their ranges northward and upslope in response to climate change. ... In the United States, some common forests types are projected to expand, such as oak-hickory; others are projected to contract, such as maple-beech-birch. Still others, such as spruce-fir, are likely to disappear from the United States altogether. ... In the Northeast, under a mid-range warming scenario, the currently dominant maple-beech-birch forest type is projected to be completely displaced by other forest types in a warmer future. ... In Alaska, vegetation changes are already underway due to warming. Tree line is shifting northward into tundra, encroaching on the habitat for many migratory birds and land animals such as caribou that depend on the open tundra landscape.
***
It is not surprising that marine species in U.S. waters are moving northward and that the timing of plankton blooms is shifting. Extensive shifts in the ranges and distributions of both warmwater and coldwater species of fish have been documented.
As warming drives changes in timing and geographic ranges for various species, it is important to note that entire communities of species do not shift intact. Rather, the range and timing of each species shifts in response to its sensitivity to climate change, its mobility, its lifespan, and the availability of the resources it needs (such as soil, moisture, food, and shelter). The speed with which species can shift their ranges is influenced by factors including their size, lifespan, and seed dispersal techniques in plants. In addition, migratory pathways must be available, such as northward flowing rivers which serve as conduits for fish. Some migratory pathways may be blocked by development and habitat fragmentation. All of these variations result in the breakup of existing ecosystems and formation of new ones, with unknown consequences.
***
The Intergovernmental Panel on Climate Change has estimated that if a warming of 3.5 to 5.5°F occurs, 20 to 30 percent of species that have been studied would be in climate zones that are far outside of their current ranges, and would therefore likely be at risk of extinction.
***
Deserts in the United States are also projected to expand to the north, east, and upward in elevation in response to projected warming and associated changes in climate.
***
Perhaps most vulnerable of all to the impacts of warming are Arctic ecosystems that rely on sea ice, which is vanishing rapidly and is projected to disappear entirely in summertime within this century. Algae that bloom on the underside of the sea ice form the base of a food web linking microscopic animals and fish to seals, whales, polar bears, and people. As the sea ice disappears, so too do these algae. The ice also provides a vital platform for ice-dependent seals (such as the ringed seal) to give birth, nurse their pups, and rest. Polar bears use the ice as a platform from which to hunt their prey. The walrus rests on the ice near the continental shelf between its dives to eat clams and other shellfish. As the ice edge retreats away from the shelves to deeper areas, there will be no clams nearby.
***
Fewer wildflowers are projected to grace the slopes of the Rocky Mountains as global warming causes earlier spring snowmelt. Larkspur, aspen fleabane, and aspen sunflower grow at an altitude of about 9,500 feet where the winter snows are deep. Once the snow melts, the flowers form buds and prepare to bloom. But warmer springs mean that the snow melts earlier, leaving the buds exposed to frost. (The percentage of buds that were frosted has doubled over the past decade.) Frost does not kill the plants, but it does make them unable to seed and reproduce, meaning there will be no next generation. Insects and other animal species depend on the flowers for food, and other species depend on those species, so the loss is likely to propagate through the food chain.
***
As precipitation increasingly falls as rain rather than snow, it feeds floods that wash away salmon eggs incubating in the streambed. Warmer water leads eggs to hatch earlier in the year, so the young are smaller and more vulnerable to predators. Warmer conditions increase the fish’s metabolism, taking energy away from growth and forcing the fish to find more food, but earlier hatching of eggs could put them out of sync with the insects they eat. ... Studies suggest that up to 40 percent of Northwest salmon populations may be lost by 2050.
Over half of the wild trout populations are likely to disappear from the southern Appalachian Mountains because of the effects of rising stream temperatures. Losses of western trout populations may exceed 60 percent in certain regions. About 90 percent of bull trout, which live in western rivers in some of the country’s most wild places, are projected to be lost due to warming. ... Projected losses of trout habitat for some warmer states, such as North Carolina and Virginia, are up to 90 percent.
***
Most wild Pacific salmon populations are extinct or imperiled in 56 percent of their historical range in the Northwest and California, and populations are down more than 90 percent in the Columbia River system. Many species are listed as either threatened or endangered under the Federal Endangered Species Act. Studies suggest that about one-third of the current habitat for the Northwest’s salmon and other coldwater fish will no longer be suitable for them by the end of this century as key temperature thresholds are exceeded.
***
Heavy rains can lead to flooding, which can cause health impacts including direct injuries as well as increased incidence of waterborne diseases due to pathogens such as Cryptosporidium and Giardia. Downpours can trigger sewage overflows, contaminating drinking water and endangering beachgoers. The consequences will be particularly severe in the roughly 770 U.S. cities and towns, including New York, Chicago, Washington DC, Milwaukee, and Philadelphia, that have “combined sewer systems;” an older design that carries storm water and sewage in the same pipes.During heavy rains, these raw sewage spills into lakes or waterways, including drinking-water supplies and places where people swim.
In 1994, the Environmental Protection Agency (EPA) established a policy that mandates that communities substantially reduce or eliminate their combined sewer overflow, but this mandate remains unfulfilled.
***
The urban heat island effect has raised average urban air temperatures by 2 to 5°F more than surrounding areas over the past 100 years, and by up to 20°F more at night.
***
In lakes with contaminated sediment, warmer water and low-oxygen conditions can more readily mobilize mercury and other persistent pollutants. In such cases, where these increasing quantities of contaminants are taken up in the aquatic food chain, there will be additional potential for health hazards for species that eat fish from the lakes, including people.
***
In the Cascade Mountains, April 1 snowpack declined by an average of 25 percent, with some areas experiencing up to 60 percent declines.
-- Global Climate Change Impacts in the United States: A State of Knowledge Report from the U.S. Global Change Research Program