2. Introduction, Data Sources, and Methodology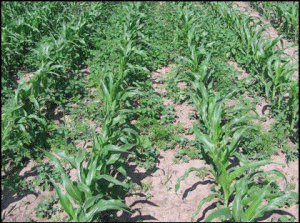
Weeds, insects, and plant diseases can significantly reduce the yield and quality of crops. Since the dawn of agriculture and around the world, managing pests has been a constant, annual, and unavoidable challenge for farmers. The effectiveness of steps taken to keep pest losses to a minimum has often meant the difference between life and death for families, tribes, communities, and even some civilizations.
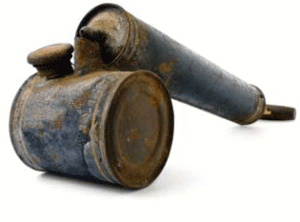
Since World War II, pesticides have become the major tool employed by U.S. farmers to combat weed competition and insect damage. The term “pesticide” encompasses any chemical designed to control, manage, or kill a pest. There are three major types of pesticides: herbicides to control weeds, insecticides to manage insects, and fungicides to control plant disease. There are several other types of pesticides including rodenticides, nematicides (nematodes), antibiotics (bacteria), plant growth regulators, and miticides (mites).
All pesticides contain one or more “active ingredients” (AI). These are the chemicals within pesticide products that are responsible for either killing a target pest outright, or undermining the ability of a target pest to thrive or do damage to a growing crop.
“Inert ingredients” are added to pesticide products to improve the efficacy and stability of a pesticide.Pesticides work through many different modes of action. Some modes of action disrupt one or more essential physiological processes within the target pest sufficiently to kill the pest in a short period of time. Other modes of action involve blocking how a pest is able to digest food, impeding growth, or impairing reproduction.
Natural biochemicals like insect pheromones (scents that attract insects), botanicals, bacteria like Bacillus thuriengensis (Bt), and horticultural oils are also classified by the Environmental Protection Agency (EPA) as “pesticides” because of their ability to help manage pests. Most of these work through a non-toxic mode of action and many are approved by the United States Department of Agriculture’s (USDA’s) National Organic Program (NOP) for use on certified organic farms.A. Tracking Pesticide Use and RiskThere are two basic ways to track changes in reliance on pesticides: first, the number of different pesticides applied on a given acre, and second, the total pounds of pesticide active ingredient applied per acre in a given year.
Pesticide use surveys carried out by the USDA (see section below on data sources for details) show that corn fields in the U.S. were treated with an average 1.07 herbicides and 0.39 insecticides in 1971, while in that year 0.72 herbicides were used on soybeans, as shown in Figure 2.1.
Figure 2.1. Corn, Soybean and Upland Cotton: Number of Herbicides Used to Manage Weeds, 1971-2008
Figure 2.2. Corn and Upland Cotton: Number of Insecticides Used to Manage Pests, 1971-2008Two decades later in 1991, corn farmers applied on average about two different herbicides per acre. Since 1991 reliance has gradually increased and reached a peak of 2.78 herbicides applied to the average acre in 2001.
Corn growers have been less reliant on insecticides than on herbicides, as clear in Figures 2.1 and 2.2. Between 29% and 39% of national corn acres have been treated with an insecticide since 1971. This lessened reliance compared to herbicides reflects two facts on the ground:
• Weeds are a problem every year in every field, while corn insects are episodic pests that cause problems serious enough to warrant treatments in only some regions and in some years; and• Planting corn and soybeans in a crop rotation is typically very effective in suppressing most important corn insect pests.Increasing reliance by soybean farmers over time on a greater number of herbicides is evident in Figure 2.1, until the introduction of Roundup Ready (RR) glyphosate-resistant soybeans in 1996. The number of herbicides applied per acre fell from 2.7 in 1996 to 1.38 in 2005, although the number of herbicides applied on soybean acres is now rising as a result of the emergence of weeds resistant to glyphosate. Very few soybean acres are treated with insecticides.
In terms of the volume, or pounds of pesticide active ingredient applied per acre, there were about
three pounds of insecticides applied in 1964 for each pound of herbicide on major U.S. field, fruit and vegetable crops.1 Just seven years later in 1971, 176 million pounds of herbicides were applied, in contrast to 128 million pounds of insecticides.Since 1971, the shift to much lower-dose insecticides has reduced the total pounds of insecticides applied to under 40 million in 2004.
Herbicide use, on the other hand, rose from 176 million pounds in 1971 to 363 million pounds in 1997, despite the registration of several lower-dose herbicides starting in the early 1980s.In 2004 across major field crops, the ERS reports that 7.6 pounds of herbicides were applied for each pound of insecticide. The unmistakable dominance of herbicides in measures of the total pounds of pesticides applied is why the performance of herbicide-tolerant GE crops determines, for the most part, the impact of GE technology on overall pesticide use.
Table 2.1 provides an overview of the acres planted and pesticide use from 1996 through 2008 for the three major GE crops: corn, soybeans, and cotton. Across these three crops and the 13 years covered in this analysis, 3.8 billion pounds of herbicides were applied, compared to 409 million pounds of insecticides – 9.3 pounds of herbicides for each pound of insecticide. Cotton is clearly an exception in that insecticide use accounts for 43% of the total pounds of pesticides applied to that crop.
Table 2.1. Corn, Soybean, and Cotton Acreage Planted, Average Pesticide Use Per Acre, and Total Pounds Applied: 1996-2008 (see notes)
Figure 2.3. Shares of Herbicides and Insecticides Applied on Corn, Soybean and Cotton: 1996-2008Environmental and public health problems with pesticides began to attract the attention of both scientists and citizens in the 1960s. Rachel Carson’s famous 1962 book
Silent Spring deepened public awareness and concern over the impact of persistent, chlorinated hydrocarbon insecticides. Government scientists and regulatory agencies focused more attention on pesticide use and risks, both confirming the existence of significant environmental impacts from pesticide use, especially insecticides, and gaining insight into how pesticides were harming birds and other wildlife, as well as people.
As pesticide use grew in the 1970s and 1980s, so did evidence of adverse impacts on exposed wildlife populations and people. The regulation of pesticide use and risks became one of the dominant areas of focus for the EPA and the environmental community in the 1980s and through much of the 1990s. An overview of pest management, pesticide use and risks, and efforts to move toward more prevention-oriented pest management systems is provided in the 1996 Consumers Union book Pest Management at the Crossroads (PMAC). 2
A key theme of PMAC is that changes in crop rotations and other farming practices can sharply reduce pest pressure and reliance on pesticides.
B. Milestones and Major Impacts of GE CropsThe application of recombinant DNA technology in crop breeding, popularly known as genetic engineering, has been promoted by the biotechnology industry as another means to reduce pesticide use. Genetically engigeered (GE) crops were introduced commercially in the U.S. in 1996 and were rapidly adopted by corn, soybean, and cotton farmers.
Table 2.2. Percent of National Acres Planted to Herbicide-Tolerant (HT) and Bt Crop Varieties [Combines acres planted to single- and multiple-trait varieties]By 1998, concern and controversy over the health and environmental impacts of GE plants had, for the most part, overshadowed long-standing worries over pesticide use and risk, both in the U.S. and Europe.
In part for this reason,
there has been surprisingly little rigorous independent analysis of the pesticide use implications of GE crop technology. This lack of solid data is all the more surprising given that: 1) nearly all commercially grown GE crops have pest management traits that directly impact pesticide use practices; and 2) the technology is being implemented and promoted by agrichemical firms that have acquired a significant share of the world’s seed supply.This report attempts to fill an important gap in understanding of the impacts of GE crop technology by answering the following question:
How have GE crops impacted pesticide use in the United States? We begin by providing brief overviews of the two major traits introduced into the three primary GE crops: herbicide tolerance and insect resistance in corn, soybeans, and cotton. GE crops with these traits comprise roughly 99% of all biotech crops grown (by acreage) in the U.S. from 1996 to 2008.3
Herbicide ToleranceHerbicide-tolerant (HT) crops are engineered to survive direct “post-emergence” application of one or more herbicides. The herbicide kills or severely stunts all or most growing weeds, while leaving the crop undamaged, or just modestly impacted for a short period of time.
A handful of HT crops was introduced prior to the advent of genetic engineering. The first such crop,
canola resistant to atrazine and related triazine herbicides, was commercialized in 1984. Interestingly, it was developed through recurrent backcrossing of canola with a related weed (Brassica campestris) from a population that had previously evolved resistance in the field through repeated application of triazine herbicides.4 Most other non-GE HT crops were developed through use of mutagenesis to be resistant to sulfonylurea and/or imidazolinone herbicides that inhibit the acetolactate synthase enzyme (ALS inhibitors). ALS inhibitor-resistant corn, soybeans, and canola were commercialized in 1992, 1994, and 1997, respectively, followed in the early years of this decade by resistant varieties of wheat, rice and sunflower.5
It is worth noting that these crops were endowed with resistance to the two classes of herbicides to which weeds, at the time, had developed the most widespread resistance, in terms of both number of resistant biotypes and acreage infested. The first major wave of herbicide resistance that began in the 1970s involved 23 species of weeds resistant to atrazine and related herbicides of the photosystem II inhibitor class, which have been reported to infest up to 1.9 million acres of cropland in the U.S. The second major wave began in the 1980s, and involves 37 species of weeds resistant to ALS inhibitors. Scientists have confirmed that these resistant weeds now infest up to 152,000 sites covering 9.9 million acres (see Figure 2.4).
Figure 2.4. U.S. Crop Acreage Infested With Herbicide-Resistant Weeds by Class of Herbicide and Year Reported: 1970-2008Compiled by Center for Food Safety from reports listed by International Survey of Herbicide Resistant Weeds (http://www.weedscience. org/In.asp), last visited Feb. 3, 2009. Acreage infested figures are reported in ranges due to the difficulty of determining the extent of a resistant weed population. The figures presented here represent aggregate upper-bound estimates. Note that glyphosate is the only member of the “glycines” class of herbicides.Though acreage figures are difficult to come by, a market research firm recently estimated that non-GE herbicide-resistant crops were planted on roughly 6 million acres in 2007.6 It was not until the advent of genetic engineering that HT crops became prevalent. This report deals only with GE HT crops.
Herbicide Resistance and Herbicide Tolerance Definitions
The following official WSSA definitions of “herbicide resistance” and “herbicide tolerance” were proposed by the Herbicide Resistant Plants Committee, accepted by the WSSA Terminology Committee, and approved the the WSSA Board of Directors at the 1998 WSSA winter Board meeting in Chicago, IL. The definitions for these terms are presented below and should be used where applicable in all future publications of the WSSA.
Herbicide resistance: “Herbicide resistance is the inherited ability of a plant to survive and reproduce following exposure to a dose of herbicide normally lethal to the wild type. In a plant, resistance may be naturally occurring or induced by such techniques as genetic engineering or selection of variants produced by tissue culture or mutagenesis.”
Herbicide tolerance: “Herbicide tolerance is the inherent ability of a species to survive and reproduce after herbicide treatment. This implies that there was no selection or genetic manipulation to make the plant tolerant; it is naturally tolerant.”
Source: Weed Technology Volume 12, Issue 4 (October-December) 1998. p. 789.
GE HT soybeans, cotton, and corn were introduced beginning in 1996 on just over 7 million acres, and their use expanded by nearly 20-fold to cover more than 132 million acres by 2008. In 2008, HT soybeans, cotton, and corn represented 92%, 93%, and 63% of total acres planted to each crop, respectively (see Figure 2.5, Table 2.2, and Supplemental Tables 2-4 for details and sources).
The vast majority of HT crops are Monsanto’s glyphosate-resistant, Roundup Ready (RR) soybeans, cotton, and corn. GE bromoxynil-tolerant (BXN) cotton was planted on modest acreage from the mid-1990s until 2004, but has since disappeared from the market (see Supplemental Table 4). The only currently grown GE crops resistant to an herbicide other than glyphosate are glufosinate-resistant cotton, corn, and canola, which are sold under the brand name
LibertyLink (LL). However, LL varieties are not widely grown, comprising no more than a few percent of U.S. cotton and corn acres. 7
["Swing the question over to the freedom-of-choice issue."]
[Stanton Glantz] The tobacco companies knew they have to appeal to something more basic than this intellectual pursuit of science. How do you do it? Do it with freedom.
[Man] That's the way freedom dies. Today it's smoking. But what will they try to regulate next?
[Man] What will they try to regulate next? What we can say? What we can read?
[Woman] I don't want Big Brother breathing down my neck telling me what to do.
[Man] Not just for smokers, but for nonsmokers,
and all others who want to live their lives ...
making their own decisions, not having them made for them by the benevolent bureaucracy of Washington wisdom, or these other --
[Stanton Glantz] By turning it into an abstract issue of freedom, and moving it away from their corporate interests,
they can get people behind it. Who can possibly be against freedom?
What happened was, these other companies, and other industries realized, like: "Hey, this is a pretty good idea."
[SMOKERS ARE CUSTOMERS TOO]
--
Merchants of Doubt, directed by Robert Kenner
Figure 2.5. Percent of National Acres Planted to Herbicide Tolerant (HT) Crop Varieties, 1996-2008A major factor driving adoption of glyphosate-resistant (GR) crops has been the declining efficacy of popular ALS inhibitors. Control problems emerged with ALS inhibitors as a result of the development of resistant weeds beginning in 1987, just five years after the first ALS inhibitor herbicide was brought to market in 1982.8 As noted above, weeds resistant to ALS inhibitors were more prevalent than any other class of herbicide-resistant weeds in the U.S.
Another reason for the dominance of RR crop systems is ease of use and the efficacy of glyphosate, an herbicide that kills a broad spectrum of weeds including annual and perennial broadleaf and grass species. RR-based cropping systems have been well received by farmers because they are simple, flexible, and forgiving.
Prior to the commercial introduction of RR HT crops, glyphosate use was restricted to either before a crop was planted or new seedlings have emerged, or after a crop was harvested. Any direct applications on a growing crop were certain to cause significant damage. RR technology widened the application window to allow post-emergence applications over the top of growing plants throughout the season, thus leading to dramatically increased use of and reliance on glyphosate-based herbicides.
As discussed further below, RR crop systems have fostered a third wave of resistant weeds that poses a serious threat to agriculture, and are also profoundly shaping the biotech industry’s product pipeline. As yet, there has been no regulatory response to the growing epidemic of GR weeds.Insect ResistanceIn contrast to herbicides, insecticide use in American agriculture has declined sharply since the mid-1960s as a result of the shift away from chlorinated hydrocarbon and carbamate insecticides applied at about one pound per acre, to synthetic pyrethroid and other insecticides applied at one-half to one-tenth pound per acre, or less.
Insect-resistant cotton and corn varieties are genetically modified to produce one or more truncated and activated forms of the toxins (e.g., Cry1Ab) derived from the soil bacterium Bacillus thuringiensis (Bt). These so-called Bt crops were introduced in 1996, and the percentage of national crop acres planted has grown rapidly, as shown in Figure 2.6.
Acreage planted to Bt crops grew from 1.8 million acres of cotton in 1996 to 55.8 million acres of corn and cotton in 2008, as shown in Supplemental Table 6.
The first Bt corn varieties, and all Bt cotton varieties, repel above-ground Lepidopteron pests such as the European corn borer (ECB), Southwestern corn borer (SWCB), and cotton bollworm. Bt corn to control corn rootworm (CRW) and other soil-borne insects was introduced in 2003.Bt toxins are biosynthesized continuously throughout the tissues of Bt plants, although genetic engineers have some ability to preferentially target (i.e., increase) expression levels in those plant tissues where the toxin is most needed to fend off insect feeding. Bt plant-incorporated toxins exert profound selection pressure for development of resistant insects by virtue of the plant’s continual production of toxin, in contrast to the intense but short-lived exposure characteristic of Bt insecticidal sprays.
The mode of action of Bt sprays and toxins is not completely known. Foliar Bt sprays contain inactive Cry protoxins (about 130-140 kDa in size) which exist in a crystalline form, when ingested. The alkaline nature of the fore- and mid-gut dissolves the crystal and cleaves it one or two times in the fore and mid-gut to create a truncated, activated toxin (about 60-65 kDa in size).
The activated Cry toxins poke a hole in the gut epithelium, but it is unclear what causes insect death. The two proposed mechanisms are: 1) disruption of the mid-gut epithelium causes insects to stop feeding and starve to death, or 2) extensive cell lysis provides the Bt access to the hemocoel, where they germinate and reproduce, leading to septicemia and death.9
Figure 2.6. Percent of National Acres Planted to Corn and Cotton Varieties, 1996-2008The toxicity of Bt sprays is limited to those insects with the alkaline gut pH required to cleave and activate the protoxin. In Bt plants, the Cry toxins are already activated, increasing the potential for adverse impacts on populations of beneficial insects.10
Even before their commercial introduction, many scientists were concerned that Bt crops would accelerate the evolution of pest resistance to Bt toxins.11
In response to such clear warnings from scientists and in the hope of delaying the emergence of resistance, the EPA mandated that Bt cotton and corn growers plant blocks of conventional (non- Bt) crop “refuges” amidst Bt fields to help slow development of resistance. Refuges work by maintaining populations of susceptible insects, some of which will mate with resistant insects, thereby diluting the presence of Bt-resistant genes in insect populations. EPA encourages “high-dose” Bt crops as another resistance management strategy; high levels of expression of Bt toxins lead to a more complete kill of target insects, and hence fewer surviving insects with the potential to pass along resistant genes.The resistant management plans imposed by EPA on Bt cotton and corn have, for the most part, been effective. However, continued vigilance is necessary, given the emergence of isolated populations of cotton bollworms resistant to Cry1Ac in Bt cotton.12
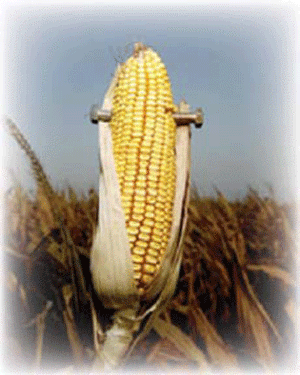
Seed companies have also begun developing Bt crops with multiple Bt toxins, both to expand the range of insects controlled and as a resistance management strategy. Bt corn with toxins for both ECB and CRW (e.g., YieldGard Plus) were introduced in 2005, and are now widely planted. Cotton with two Bt toxins (Bollgard II) was introduced in 2003, and
SmartStax corn varieties will be sold for the first time in 2010 expressing six different Bt toxins, three for the ECB and SWCB, and three more for the CRW.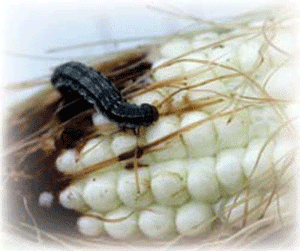
New issues arise in assessing risks associated with the stacked versions of crops that have more than one Cry protein.
There may be a synergistic effect between the various Cry proteins which could affect the efficacy of the various Cry proteins against their target and non-target organisms. Cross-resistance could emerge as a new challenge in managing resistance.
Additional data will also be needed for human toxicity and environmental effects.13 For instance, the EPA recently funded research to develop an animal model of allergenicity to better assess the potential for Bt insecticidal proteins to trigger food allergies.14
C. Data Sources and ComplicationsThis report is based on surveys of agricultural chemical use conducted by USDA’s National Agricultural Statistics Service (NASS). We chose to base this analysis on USDA data for several reasons. First, NASS supplies highly reliable data through use of transparent, rigorous methods and statistically representative sampling procedures.15 Second, because the NASS program has collected annual pesticide usage data on soybeans, corn, and cotton for most of the years covered by this report, it offers a consistent dataset that facilitates accurate, year-to-year comparisons. Finally, the public availability of NASS data (free of charge) facilitates open review and criticism of any analysis utilizing them.


NASS data are considered the gold standard of pesticide use information in the U.S. NASS reports provide a solid basis to study trends in the intensity of pesticide use across crops and regions, among pesticide families of chemistry, and over time. These reports are a valuable resource used by EPA and state pesticide regulatory agencies, farm commodity groups, the food industry, environmental and consumer groups, and the pesticide industry.
Several private firms16 also collect pesticide use information, under contract with mostly corporate subscribers, such as agrichemical companies. These sources are unacceptable for use in this report for several reasons, including their great expense, the proprietary nature of sampling methodologies, and prohibitions on the use and/or disclosure of purchased data.17
Because USDA does not routinely collect separate data for pesticide use on GE and conventional crops, a methodology is needed to estimate average pesticide use on GE and conventional crop acres. Such a methodology was first developed in 2003 and used in the analysis reported in Ag BioTech InfoNet Technical Paper #6, “Impacts of Genetically Engineered Crops on Pesticide Use in the United States: The First Eight Years.”
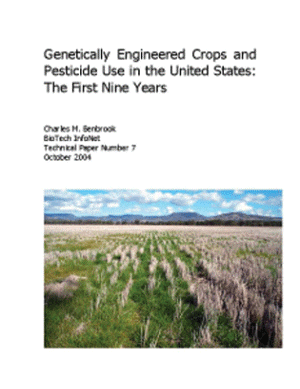
The method was refined and applied to an additional year of USDA pesticide use data in the October 2004 Ag BioTech InfoNet report “Genetically Engineered Crops and Pesticide Use in the United States: The First Nine Years.”18 The same basic approach has been applied in this analysis covering the first 13 years of commercial planting of GE crops.
USDA has surveyed pesticide use for five decades beginning in 1964. Subsequent national surveys were conducted in 1966, 1971, and 1982. These early surveys covered only a few major crops and collected just basic data like the percentage of acres treated and pounds of active ingredient applied.
From 1991 through 2001, NASS surveyed pesticide use on major field crops including corn, soybeans, and cotton on an annual basis. Annual summary reports have been issued with a set of tables covering pesticide use in all “Program States,”19 as well as at the national level.
Each standard table for a given crop reports the percentage of acres treated with a specific pesticide active ingredient, the average rate of application in pounds of active ingredient per acre; the average number of applications; the average rate per crop year, which is simply the one-time application rate multiplied by the number of applications; and the total pounds applied.
Benbrook Consulting Services (BCS) and Ecologic, Inc. have moved NASS survey data into a database program to carry out additional computations. For instance, average figures for individual and aggregate pesticide use in the Program States are applied to the small proportion of acres that NASS does not survey to arrive at estimates of total pesticide use for all crop acres in any given year.20
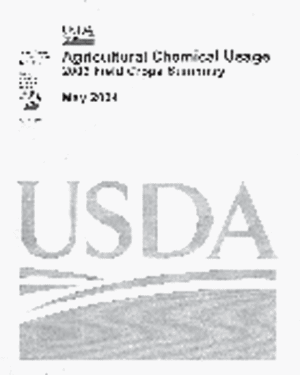
In years when a given crop was not surveyed by NASS (e.g., cotton in 2006), average values are interpolated between the previous and following year to fill in such data gaps. For corn since 2005, soybeans since 2006, and cotton for 2008, herbicide and insecticide use rates were projected from recent trends and in light of published reports on university websites regarding levels of pest pressure and the emergence of resistant weeds or insects.
Spikes upward in pesticide use are readily apparent in NASS data and have alerted farmers, scientists, and USDA to pest-induced problems in specific crops and regions. Such problems might be triggered by the emergence of resistance to a once-effective pesticide or the introduction of a new invasive species. Likewise, reductions in the frequency and intensity of pesticide use are regarded as evidence that farmers have made progress in adopting prevention-based Integrated Pest Management (IPM), perhaps through the planting of a new crop variety or adoption of a more complex crop rotation.
By combining NASS pesticide use data with EPA data on the toxicological potency of pesticide active ingredients, pesticide risk indices specific to different classes of organisms, like birds or bees, have been calculated by the Economic Research Service (ERS) and other analysts. Such indices provide a useful early-warning system to detect changes in pest pressure, or pesticide efficacy over time and in different regions that may lead to “unreasonable adverse effects on man or the environment,” the basic standard embedded in U.S. pesticide regulatory policy.
Scientists studying the emergence of resistance to a specific pesticide, or family of chemicals, rely heavily on pesticide-use data to determine the degree of selection pressure required to trigger resistance.21 Epidemiologists exploring associations between pesticide use, exposure and patterns in birth defects or cancer often use NASS data in constructing retrospective estimates of exposure levels.
Impacts of USDA Decision to Stop Collecting Pesticide Use DataNASS has dramatically scaled back its program in recent years. First, NASS replaced its annual surveys of major field crops with less frequent ones beginning in 2002. Then, in the 2007 growing season, data collection was limited to just two crops—cotton and apples. NASS did not collect pesticide use data on any crops during the 2008 growing season, citing a shortage of funds and the availability of private sector survey data as reasons for cutting the program.22Of the three major crops covered in this report, NASS data are available in most years for cotton through 2007, through 2006 for soybeans, and through 2005 for corn.
The absence of a continuous series of NASS data since 2005 for the three major GE crops hampers the ability of independent analysts and government scientists to track the performance and impacts of GE crops. The lack of NASS pesticide-use data covering recent crop years is a special concern, given the dramatic impact of resistant weeds on the number and volume of herbicides applied to HT crops.USDA’s decision to drop the pesticide-use surveys led to strong protests from a wide range of groups, including The Organic Center, Center for Food Safety, Union of Concerned Scientists, Natural Resources Defense Council, and many other organizations, including several with close ties to the pesticide industry.23 In 2008, the administrator of the EPA voiced concern to the Secretary of Agriculture about the loss of NASS data, joining several government officials at the state and federal levels.
In May, 2009, the new USDA leadership announced the reinstatement of the program, beginning with the fruit and nut survey in the fall of 2009.24
D. MethodologyIn this report a four-step methodology is used to calculate the differences in the amount of pesticides applied to GE crops versus conventional crops in a given year.
First, the total number of acres of each crop planted to conventional, HT and/or Bt varieties is derived from standard USDA sources: NASS for soybeans and corn, the Agricultural Marketing Service (AMS) for cotton.
Monsanto’s “Biotechnology Trait Acreage” reports are used to disaggregate total Bt corn trait acres to those planted to varieties engineered to control the ECB, the CRW, or both.
Second, the average amount of pesticides applied per acre per crop year is estimated for conventional GE crop acreage (detailed results in Supplemental Table 7).
Third and by year, the average amount of herbicides or insecticides applied to an acre planted to a conventional seed variety is subtracted from the corresponding amount for the GE crop.
Finally, in the fourth step, the difference in pesticide pounds applied per acre for each GE trait is multiplied by the acres planted to the GE crop in that year (full results appear in Supplemental Table 8). The impacts of herbicide tolerant and Bt crops on pesticide use per acre are then added together across the three crops over the 13 years of commercial use, producing the overall impact of today’s major GE crops on herbicide, insecticide, and all pesticide use.
Estimating Herbicide Application Rates on Conventional and HT Soybeans, Corn, and CottonBecause the USDA does not report herbicide-use data separately on acres planted to conventional varieties, in contrast to GE varieties, an indirect method was developed that draws on NASS data. The method involves the use of a standard formula to estimate what is not known, from variables that are known from NASS and other data sources.
The average pounds of herbicides applied on all corn, soybean, or cotton acres in a given year are easily calculated from NASS data. Data are readily accessible on the share of total crop acres in a given year that were planted to conventional crop varieties, as well as the percentage planted to GE varieties. These two percentages add up to 100% and can be used in a weighted-average formula, along with average herbicide use on GE crop acres, to calculate the pounds of herbicides applied on non-HT acres.
The basic weighted average formula, as applied to the pounds of herbicides used in producing HT and conventional acres of cropx, contains the following five data elements, the first four of which are known or can be projected from USDA.
1. Average herbicide use per acre on all acres planted to a crop, from NASS surveys;
2. The percentage of acres planted to HT crops, from ERS and AMS data;
3. The percentage of acres planted to conventional varieties (100% minus number 2);
4. The average pounds of all herbicides applied per acre of HT crop, from NASS surveys and university sources; and
5. The average pounds of herbicides applied per acre of conventional crop, which can be calculated by solving the weighted-average equation for the variable “Ave. Pounds Applied on non-HT acres cropx.”25
The basic formula is:
Average Herbicide Pounds Applied per Acre on All Acres of cropx = (% acres planted to HT varieties cropx x Ave. Pounds Applied on HT varieties cropx) + (% acres planted non-HT varieties cropx x Ave. Pounds Applied on non-HT acres cropx)
For a given crop and year, we calculated the impact of HT technology on herbicide use by subtracting the average rate applied to conventional acres (number 5 in above list) from the average rate applied to HT acres (number 4 in above list). When this number is negative, HT technology reduced herbicide use in that year for that crop; when it is positive, average herbicide use was higher on HT acres.
Insecticide Application Rates on Conventional and Bt Corn and Cotton AcresIn the case of Bt corn, two steps are required to estimate the impact of an acre planted to Bt corn for ECB/SWCB and/or CRW control on corn insecticide use. First, the average rate of application per crop year must be calculated for insecticides targeting the ECB and the CRW. This process is complicated by the fact that several insecticides are applied for control of both the ECB and CRW. For these insecticides, the portion of acres treated for control of ECB versus the CRW must be estimated. We reviewed pesticide labels, treatments recommended in university spray guides, and consulted with experts in corn IPM in carrying out this step (see Supplemental Table 9 for the share of insecticide acres treated targeting the ECB and Supplemental Table 10 for the share targeting the CRW).
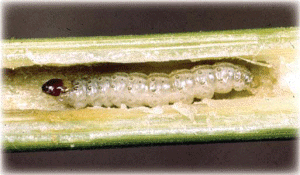
The percentage of national corn acres treated with each insecticide for ECB/SWCB and CRW control was used to calculate a weighted average rate of insecticide application across all corn acres treated per crop year. Based on these calculations, the weighted average rate of insecticides applied on conventional acres for ECB control drops from 0.2 pounds of active ingredient per acre in 1996 to 0.15 in 2005-2008. In the case of CRW, the rate of insecticides applied on conventional acres falls from 0.29 pounds per acre in 2003, the year Bt corn for CRW control was commercialized, to 0.19 pounds in 2005-2008. Figure 2.7 shows the weighted-average rate of application for insecticides targeting the ECB and CRW.
The second step in calculating the pounds of insecticides displaced by the planting of Bt corn is to estimate the portion of acreage planted to Bt corn for ECB and/or CRW control that would have been treated with an insecticide if the corresponding Bt crop had not been planted. This step is required since Bt corn is now planted on far more acres than were ever treated with insecticides. Historically, USDA data show that before the advent of Bt corn, just 6% - 9% of national corn acres were typically treated for ECB/SWCB control, while 27% +/- 4% were treated for CRW control.
Supplemental Table 11 provides the details of this step and the resulting estimates of insecticide use averted through the planting of Bt corn for ECB and/or CRW control.
In the case of Bt acres targeting the ECB/SWCB, the likely share of acres planted to Bt corn that would have been sprayed for ECB control begins at 90% in 1997, the first year of commercial planting, and drops incrementally to 45% in 2008, a year when over half of corn acres were planted to a Bt corn variety engineered for ECB control.
The high initial percentage is based on the assumption that early adopters of Bt ECB corn were more likely to have been farmers contending with serious ECB and/or SWCB infestations, triggering the need for insecticide applications. The falling percentage reflects the progressively wide adoption of Bt corn by farmers with lesser ECB/SWCB problems, many of whom likely did not spray prior to the commercial launch of Bt corn.
In the case of Bt corn for CRW control, the percentage of acres planted that would likely have been treated with an insecticide targeting the CRW begins at 95% in 2003, the first year of commercial sales, and declines to 60% in 2008, a year when 35% of corn acres were planted to a Bt corn for CRW control and another 9% of corn acres were sprayed for CRW control with an insecticide (i.e., about 44% of corn acres were either sprayed or planted to a Bt variety for CRW control, well above the 27% +/- 4% level treated with insecticide for CRW from 1964 through 2008).
Figure 2.7. Insecticide Use Rates to Control ECB and CRW, 1996-2008This higher projected level of CRW treatment of corn acres is justified in part by the emergence in the late 1990s of a variant of the CRW that learned to overwinter in soybean fields, thus undermining the efficacy of corn-soybean rotations in reducing CRW populations.
Bt cotton targets the budworm/bollworm complex, but does not appear to have significant effects on other insect pests, including the boll weevil, plant bugs, white flies, and stink bugs. Growers typically apply broad-spectrum insecticides to control both the budworm/bollworm complex and other insects. Bt cotton will reduce the use of insecticides for budworm/bollworm complex, but not applications of insecticides targeting other insects.
Supplemental Table 12 reports the basis for estimating the pounds of insecticides averted by each acre planted to Bt cotton. First, university insect management guides and experts were consulted to estimate the portion of total acres treated with each cotton insecticide for control of the budworm/bollworm complex versus other insects. Then the number of acres treated with each insecticide is calculated from NASS data, as well as the share of total acres treated that was accounted for by a given insecticide. Finally, weighted average use rates were calculated using the shares of total acre treatments with each individual insecticide.
In the case of cotton, this weighted average insecticide application rate falls modestly from 0.56 pounds per acre in 1996 to 0.47 in 2007-2008.
E. Assumptions and CaveatsThe methodologies used to project pesticide use on conventional and GE-crop acres require a number of assumptions and projections. Here, a brief description is provided of the major assumptions embedded in the Supplemental Tables that form the operating core of the model used to estimate the impact of GE crops on pesticide use. Each assumption or projection is also assessed in terms of its impact on our analysis of pesticide-use levels.
1. Farmers planting GE-crop varieties take advantage of the novel traits they are paying for.
For example, in the case of herbicide-tolerant plants, it is assumed that farmers build their weed management program around glyphosate herbicide. Likewise, a farmer purchasing a stackedtrait corn or cotton variety will alter both weed and insect pest management systems in accord with the purchased traits.
These assumptions closely reflect reality up to the 2009 crop season, but may not in the future as the seed industry moves toward more multiple-trait stacked varieties.
2. A small acreage of corn and cotton planted to GE herbicidetolerant varieties other than those resistant to Roundup are included in the herbicide-tolerant acreage estimates from the NASS and AMS. Herbicide use on these non-RR acres, however, is analyzed as if the acres were planted to a RR variety.
Perhaps 15 million acres have been planted to non-RR HT varieties over the last 13 years, a period during which approximately 941 million acres of RR crops have been planted. Accordingly, these non-RR HT acres account for just one out of every 63 acres of HT crops. In addition, the differences in herbicide use on non- RR HT crops, compared to RR crops, are modest. As a result, this assumption has virtually no impact on the outcome of the analysis.
3. Bt-crop growers apply no chemical insecticides for the pests targeted by these trait(s): ECB/SWCB and CRW, and the budworm/bollworm complex.
This assumption assumes close to 100% control of target pests, and overstates efficacy in regions with high pest pressure, especially where multiple generations of target pests are common. As a result, the displacement of insecticide use is likely overstated in the case of some acres planted to Bt crops. For example, University of Illinois entomologists have documented spotty performance of Bt corn for CRW control, especially under high population pressure, and reported that some growers have applied soil insecticides on Bt-corn acres.26
In fact, there was so much farm press media attention on the benefits of applying a soil insecticide on corn acres planted to a Bt corn for CRW control that the top entomologists in the University of Illinois felt compelled to ask – and answer “No” to - the following question in a widely read bulletin for growers:
“Does it always make sense to use a soil insecticide in conjunction with a Bt [CRW] Hybrid?”27
Accordingly, this assumption overstates the reduction in insecticide use on some Bt corn acres. But because corn insecticides are applied at relatively low rates, the impact of this assumption is modest. This could change dramatically, of course, if resistance emerges to the Bt toxins engineered into corn for CRW control, and farmers are forced to apply higher-rate insecticides to prevent serious CRW feeding damage.
4. It is possible to estimate the shares of the pounds applied of a given, broad-spectrum insecticide across multiple target insects, so that these shares can be used in estimating the rate of insecticide applications displaced by a given Bt trait.
Bt varieties have many complex impacts on insect communities and populations. In some fields, lessened insecticide use allows secondary pests to reach damage thresholds, triggering the need for additional insecticide sprays.28 In other fields or perhaps in certain years, the reduction in insecticides targeting key Lepidopteron insects creates an opening for populations of beneficial insects, like assassin bugs, to expand, increasing the effectiveness of biological control, and reducing the need for insecticides.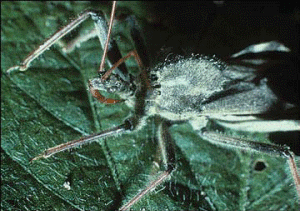
Several different species of assassin bugs attack the large larval stages of lepidopteran insects (e.g., bollworm).
Several broadspectrum insecticides applied by corn and cotton growers help manage multiple insects, including some which are, and others which are not, the target of the Bt toxins engineered into Bt corn and cotton varieties. Thus, crediting Bt corn for ECB/SWCB control with displacement of all the pounds of organophosphate or synthetic pyrethroid insecticides applied would overstate the impacts of the technology, since a portion of most of these insecticides are applied by farmers for the control of other insects, including the CRW. Th rough consultation with insect pest management guides and entomologists, these shares were approximated for the key target pests of Bt-crop varieties. In some cases the shares used in the model likely overestimate displacement, while in others, displacement is likely underestimated. Given that most insecticides now applied to corn and cotton acres are low-dose products, discrepancies in these shares will have a modest impact on the pounds of insecticides displaced by Bt crops, especially relative to changes in the pounds of herbicides applied on HT acres.
5. Some portion of the acres planted to Bt corn do not displace insecticides because before the commercial availability of Bt-corn seed, farmers were not treating their fields with insecticides.
Historically, around 35% +/- 4% of corn acres have been treated each year with an insecticide for control of the ECB, SWCB, CRW, and other insect pests. In 2008, 57% of corn acres were planted to a Bt variety, including many acres planted to a dual-Bt variety. For this reason, crediting each acre of corn planted to a single Bt trait with displacement of an insecticide acre treatment would substantially overestimate the reduction in insecticide use attributed to the technology.
As previously noted, corn insect pressure, however, has also changed in recent years as a result of the emergence of a new subspecies of the CRW that overwinters in soybean fields and disrupts the efficacy of the corn-soybean rotation in reducing CRW populations.
This variant of the CRW was taken into account by increasing the share of Bt-corn acres assumed to displace insecticide applications to well above historic levels of insecticide use. The projections of Bt corn impacts on insecticide use reflect a near doubling of the percentage of acres that farmers would likely spray with an insecticide, in the absence of Bt corn.
Mike Gray, Extension entomologist at the University of Illinois, examines a trap for the presence of CRW beetles in a soybean field.This assumption likely leads to a modest overestimate of the displacement of insecticide use caused by Bt corn, since corn farmers have other proven alternatives to reduce CRW populations through IPM systems. Regrettably, some corn farmers have lost interest in the multi-tactic approaches used in successful IPM systems as one consequence of the planting of Bt corn.
6. The Bt toxins manufactured within the cells of Bt crops are not counted as insecticides “applied” on Bt-crop acres.
Clearly, this assumption underestimates the pounds of insecticidal compounds required to manage insects on Bt crop acres. Opinions differ among entomologists, the industry, and other experts on whether it is appropriate to count Bt toxins manufactured inside GE plants as equivalent to a liquid Bt insecticide sprayed on the outside of the plant.
Uncertainty over the exact mode of action of Bt insecticides and GE toxins is part of the reason for differing opinions.Those who argue that plant-manufactured Bt toxins should not count as equivalent to an applied insecticide assert that a Bt variety is just like any other new plant variety that has been bred to express some plant protein or phytochemical useful in combating insect-feeding damage.
Those skeptical of this position point to major differences in the two Bt delivery systems and in the source of the Bt toxin. Bt liquid sprays are applied only when and as needed, consistent with the core principles of IPM. Liquid sprays expose pest populations to short-lived selection pressure, thereby reducing the risk of resistance.
Bt plants, however, produce the toxin continuously during the growing season, not just when needed, and in nearly all plant tissues, not just where the toxins are needed to control attacking insects. In a year with low pest pressure, farmers can decide not to spray insecticides on a corn field, but they cannot stop Bt hybrids from manufacturing Bt toxins in nearly all plant cells.29There is another key difference that rarely is acknowledged. When plant breeders develop a new variety with a higher level of resistance to a given insect through traditional breeding techniques, they do so by selecting a top-yielding variety to crossbreed with another variety that expresses relatively higher levels of natural phytochemicals that discourage pest feeding, disrupt pest development or reproduction, or in some way reduce the viability of pest populations.
It is extremely rare for a new crop variety developed through conventional breeding to reduce insect feeding damage by killing the target insects. Instead, the elevated levels of phytochemicals in the new variety work through one or more non-toxic modes of action.
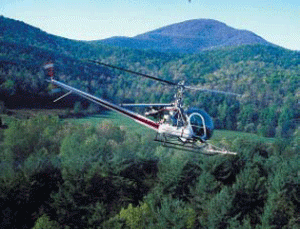
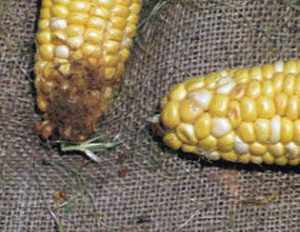
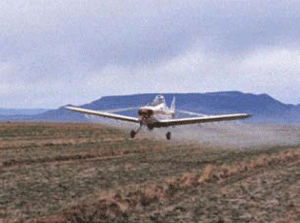
This is a second reason why some entomologists reject the notion that there is nothing different between a crop variety genetically engineered to synthesize Bt toxins within plant cells, and a new variety from conventional breeders that has improved resistance to an insect pest because of altered levels of natural phytochemicals that work through a non-toxic mode of action.
No resolution is in sight for this complex debate within the entomological community. In addition, no method exists to estimate the pounds of Bt toxins produced by a corn or cotton plant during a growing season. Hence, there is no way to project the pounds of Bt produced by an acre of Bt corn or cotton. Work is needed to develop such a methodology.
It will likely show that there is a surprisingly large amount of toxin synthesized by plants during a typical growing season, especially in the new corn varieties engineered to produce six Bt toxins.7. The last NASS survey of soybean herbicide use was in 2006. Glyphosate application rates per crop year on soybeans are projected to increase 5% annually from 2006-2007 and from 2007-2008. Cotton was surveyed last in 2007, and the glyphosate rate was projected to increase 10% from 2007- 2008. Corn was last surveyed in 2005, and the glyphosate and total herbicide rates per crop year are projected to increase 5% annually since 2005.
These assumptions are likely conservative in the case of soybeans and cotton. In soybeans, the glyphosate rate of application per crop year rose 9.8% annually from 1996 through 2006 – almost twice the rate of increase projected in 2007 and 2008.
In cotton, the glyphosate rate per crop year rose 18.2% annually from 1996 through 2007, again well above the 10% increase incorporated in the model’s projections of herbicide use on HT cotton acres in 2008.
The corn herbicide rate projections are the most uncertain, given that NASS last surveyed corn in 2005. The percentage of corn acres planted to HT varieties rose from 15% to 26% between 2003 and 2005. In this period, the rate of glyphosate applied per crop year rose on average 7.1% per year. Accordingly, the projected increase of 5% annually in the glyphosate rate per crop year in 2006, 2007, and 2008 is likely conservative. Plus, HT corn has been in widespread use now for about five years – long enough for weed shifts and resistance to begin pushing application rates per crop year upward more sharply than in the first few years of widespread commercial use.
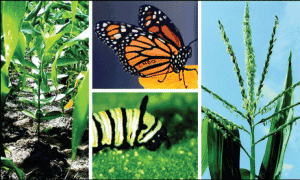
_______________
Notes:1 “Agricultural Resources and Environmental Indicators, 2006 Edition,” edited by Wiebe, K., and Gollehon, N., Economic Research Service (ERS) Information Bulletin Number 16, USDA, July 2006.
2 Benbrook, C., Groth, E., Halloran, J., Hansen, M., Marquardt, S., (1996). Pest Management at the Crossroads (PMAC), Consumers Union, PMAC also discusses the likely impacts and problems associated with GE crops, based on what was then known about the technologies.
3 GE canola has been planted on no more than 1 million acres annually; GE papaya is grown only in Hawaii on roughly 1,000 acres (and nowhere else in the world); the acreage of GE squash is unknown but almost certainly miniscule. GE sugar beets were not planted on a commercial scale until 2009.
4 Tranel, P. J., and Horvath, D. P., (2009). “Molecular biology and genomics: new tools for weed science,” Bioscience 59(3): 207-215, p. 208.
5 Tranel and Horvath (2009), op. cit., Table 1.
6 Doane Market Research and Biotech Traits Commercialized: Outlook 2010, as cited in USDA APHIS (2008). “Finding of No Significant Impact on Petition for Nonregulated Status for Pioneer Soybean DP-356043-5,” USDA’s Animal and Plant Health Inspection Service, July 15, 2008, Response to Comments, p. 26.
http://www.aphis.usda.gov/brs/aphisdocs ... 1p_com.pdf7 For LibertyLink cotton, see Supplemental Table 4; for LibertyLink corn, see Chapter 4(B).
8 Tranel, P. J., and Wright, (2002.), “Resistance of weeds to ALS-inhibiting herbicides: what have we learned?” Weed Science 50:700-712.
9 Broderick, N.A. et al (2006). “Mid-gut bacteria required for Bacillus thuringiensis insecticidal activity.” Proceedings of the National Academy of Sciences, 103(41): 15196-15199.
10 For more on Bt modes of action, including differences re: target and non-target species, see: Then, C. (2009), “Risk assessment of toxins derived from Bacillus thuringiensis—synergism, efficacy, and selectivity,” Environ Sci Pollut Res, Access at:
http://www.springerlink. com/content/a42th8677132802g/fulltext.pdf Published online June 26, 2009.
11 Harris, M. K. (1991). “Bacillus thuriengensis and Pest Control,” Letter to Science, Vol. 253, September 6.
12 Tabashnik, B.E., et al (2008). “Insect resistance to Bt crops: evidence versus theory,” Nature Biotechnology 26(2): 199-202.
13 For a recent report on additional data needs for Bt proteins, see
http://www.epa.gov/scipoly/sap/meetings ... y/022526fi nalreport.pdf
14 EPA (2009). “EPA grant to University of Chicago for research on food allergy triggers,” EPA Press Release, at
http://www.epa.gov/ ncer/events/news/2009/07_28_09_feature.html July 23.
15 USDA NASS (2006). “Meeting of the Advisory Committee on Agriculture Statistics (ACAS): Summary and Recommendations,” USDA National Agricultural Statistics Service, Appendix III, at:
http://www.nass.usda.gov/About_NASS/Advisory_Committee_ on_Agriculture_Statistics/advisory-es021406.pdf February 14-15, 2006.
16 For instance, Doane Marketing Research and Crop Data Management Systems.
17 USDA NASS (2006), op. cit.
18 Access this 2004 report at
http://www.organic-center.org/science. latest.php?action=view&report_id=158
19 “Program States” are those surveyed that year by NASS, and typically represent 85% or more of the national acreage planted to a given crop.
20 This is accepted practice, e.g. see “Agricultural Resources and Environmental Indicators: Pest Management Practices,” USDA Economic Research Service, Report No. AH722, September 2000, Table 4.3.1, footnote 1, accessible at
http://www.ers.usda. gov/publications/arei/ah722/arei4_3/DBGen.htm. “The estimates assume that pesticide use on acreage in non-surveyed States occurred at the same average rate as in the surveyed States.”
21 For instance, see: Owen, M. D. K., and Zelaya, I. A., (2005). “Herbicide-resistant crops and weed resistance to herbicides,” Pest Manag Sci 61: 301-311.
22 Engelhaupt, E. (2008). “Government pesticide and fertilizer data dropped,” Environ. Sci. Techol. 42(18), 6779-6780, at:
http://pubs. acs.org/doi/pdfplus/10.1021/es801937k?cookieSet=1.
23 For a press release with a link to the letter from 44 organizations to former Secretary of Agriculture Ed Schafer, see
http://truefoodnow. wordpress.com/fi les/2009/10/usda-nass-pr-fi nal-without-hyperlinks. doc.
24 Letter of May 7, 2009 from Katherine Smith, Acting Deputy Under Secretary for Research, Education, and Economics, to Dr. Charles Benbrook, The Organic Center.
25 The weighted-average formula can be used to calculate average herbicide use on conventional crop acres by subtracting the term (% acres planted to HT varieties cropx x Average Pounds Applied on GE varieties cropx) from both sides of the equation, and then dividing by the percentage of crop acres planted to non-GE varieties.
26 Steffey, K., (2007). “Bt Corn + Soil Insecticide: What?”, The Bulletin, University of Illinois Extension, No. 23, Article 4, October 5.
27 “Preliminary Node-Injury Ratings from University of Illinois Rootworm Product Efficacy Trials Near DeKalb, Monmouth, Perry, and Urbana,” The Bulletin, University of Illinois Extension, No. 23, Article 3, October 3, 2008.
28 Caldwell, D. (2002), “A Cotton Conundrum,” Perspectives, OnLine: The Magazine of the College of Agriculture and Life Sciences, North Carolina State University,
http://www.cals.ncsu. edu/agcomm/magazine/winter02/cotton.htm Winter 2002.
29
Moreover, from a food safety perspective, Bt toxins in liquid sprays break down relatively quickly in the field when exposed to sunlight and hence do not end up in the harvested portion of crops. Bt toxins in GE plants are inside plant cells, including the cells of the harvested portion of the crop fed to animals or consumed by people.